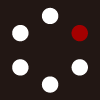
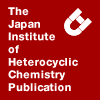
HETEROCYCLES
An International Journal for Reviews and Communications in Heterocyclic ChemistryWeb Edition ISSN: 1881-0942
Published online by The Japan Institute of Heterocyclic Chemistry
e-Journal
Full Text HTML
Received, 15th April, 2011, Accepted, 17th June, 2011, Published online, 29th June, 2011.
DOI: 10.3987/COM-11-S(P)12
■ Natural Products-Based Insecticidal Agents 8. Design, Semisynthesis and Insecticidal Activity of Novel O-(Deoxypodophyllotoxin-4’-yl)-(N-((un)substituted benzyl)indol-3-yl)glyoxylesters against Mythimna separata Walker
Yi Wang and Hui Xu*
Laboratory of Pharmaceutical Design & Synthesis, College of Life Sciences, Northwest A & F University, Yangling, Shaanxi, 712100, China
Abstract
A series of novel O-(deoxypodophyllotoxin-4′-yl)-(N-((un)substituted benzyl)indol-3-yl)glyoxylesters (8a-j) were prepared and their insecticidal activities were evaluated against the pre-third-instar larvae of Mythimna separata Walker in vivo at the concentration of 1 mg/mL. Compounds 8a, 8e-g, and 8i exhibited more potent insecticidal activity than or comparable to toosendanin, a commercial insecticide derived from Melia azedarach. Generally, it obviously suggested that the methyl group at the 6-position on the indolyl ring, the chlorine atom at the 3-position on the benzyl moiety, and introduction of the substituent on the hydroxyl group at the C-4′ position of 4′-demethyl-4-deoxypodophyllotoxin were important for the insecticidal activity.INTRODUCTION
Although the routine use of a wide variety of synthetic insecticides in agriculture has now become an accepted practice, the application of those agrochemicals over the years has resulted in the development of resistance in insect pest populations and environmental problems. Plant secondary metabolites result from the interaction between plants and environment (life and non-life) during the long period of evolution in plants, therefore, the discovery and development of new insecticidal compounds from plant secondary metabolites, followed by using them as the lead-compounds for further modifications has been one of the important ways for research and development of new pesticides in recent years.1
Podophyllotoxin (1, Figure 1), one of the well-known naturally occurring aryltetralin lignans, is extracted as the main component from the roots and rhizomes of Podophyllum species such as P. hexandrum and P. peltatum, and has been used as the lead-compund for synthesis of potent anticancer agents, such as etoposide, teniposide and etopophos. However, their potential therapeutic applications are often hindered by the development of drug-resistance, myelosuppression and cytotoxicity towards normal cells. Recently, extensive structural modifications on 1 to develop new agents to overcome the aforementioned problems and improve antitumor activity have been carried out.2-4 On the other hand, compound 1 also exhibited the interesting insecticidal activity.5-8 To obtain compounds with better insecticidal activity, more recently, a series of podophyllotoxin derivatives, such as 4′-aromatic esters (3, Figure 1) and substituted benzenesulfonates (4, Figure 1) of 4-deoxypodophyllotoxin (2, Figure 1),9,10 have been studied in our research group, and some compounds exhibited more promising and pronounced insecticidal activity than toosendanin, a commercial insecticide derived from Melia azedarach. In the meantime, indibulin (II, Figure 2), N-(pyridin-4-yl)-(1-(p-chlorobenzyl)indol-3-yl)glyoxylamide, was identified as a potent tubulin inhibitor.11 Based upon the aforementioned observations, and in continuation of our program aimed at the discovery and development of natural products-based compounds as insecticidal agents,8-10 consequently, in this paper we wanted to design and prepare a series of novel O-(deoxypodophyllotoxin-4′-yl)-(N-((un)substituted benzyl)indol-3-yl)glyoxylesters (8a-j, Figure 2) as the insecticidal agent by combining the 4′-demethyl-4-deoxypodophyllotoxin fragment (I, Figure 2) with the indolyl glyoxal moiety. Additionally, the structure-activity relationship (SAR) of these compounds were preliminarily investigated.
RESULTS AND DISCUSSION
As outlined in Scheme 1, 1-benzylindoles (6a-j) were prepared in 61-97% yields from indoles and benzyl chlorides in the presence of NaOH, KI, and triethylbenzylammonium chloride (TEBA) in dried dichloromethane (DCM). Subsequently, to a solution of oxalyl chloride in dried DCM at 0 °C under N2, a solution of 6a-j in dried DCM was added dropwise. When the reaction was complete after 2-3 h, the solvent and the excess oxalyl chloride were removed under the reduced pressure to give the intermediates 7a-j, which were used directly for the next step without further purification. Meanwhile, 4′-demethyl-4-deoxypodophyllotoxin (5) was synthesized from podophyllotoxin (1) by catalytic hydrogenolysis in the presence of 10% palladium/carbon, followed by regioselective 4′-demethylation of 4-deoxypodophyllotoxin (2) with dry hydrogen bromide.9 Finally, ten novel O-(deoxypodophyllotoxin-4′-yl)-(N-((un)substituted benzyl)indol-3-yl)glyoxylesters (8a-j) were obtained in 55-86% yields by the reaction of 7a-j with 5 in the presence of triethylamine (Et3N) at 0-5 °C. The structures of all target molecules 8a-j were well characterized by 1H NMR, MS, HRMS, optical rotation, IR, and mp.
The insecticidal activity of compounds 5 and 8a-j against the pre-third-instar larvae of M. separata was tested at the concentration of 1 mg/mL by leaf-dipping method.9 Toosendanin, a commercial insecticide derived from M. azedarach, was used as a positive control at 1 mg/mL. The corrected mortality rates of M. separata caused by 5 and 8a-j with the advance of time were described in Figure 3. The corresponding mortality rates after 35 d were far higher than those after 10 and 20 d. Especially the corresponding mortality rates of 8a-j increased sharply during 25 d to 35 d, e.g., at the stage from pupation to adulthood. That is, these compounds showed delayed insecticidal activity. For example, the corrected mortality rate of 8a against M. separata after 10 d was only 3.3%, after 20 d the corresponding mortality rate was increased to 20%, but after 35 d the corresponding mortality rate was rapidly increased to 55.5%, which was more than 16 times of that after 10 d (Table 1).
Meanwhile, the symptoms of the tested M. separata were also characterized by the same way as our previous reports. The pupation of the larvae and the adult emergence of M. separata were inhibited by these compounds, therefore, the stage from the larvae to adulthood of M. separata was prolonged as compared with the control group. Moreover, many larvae of the treated groups moulted to abnormal pupae, which could not reach adulthood and died during the stage of pupation because they were not able to remove their pupal skin. Malformed moths with imperfect wings were also found. The typical bioassay pictures of 8f and 8j were depicted in Figure 4.
As shown in Table 1, compounds 8a, 8e-g, and 8i exhibited more potent insecticidal activity than or comparable to toosendanin. Meanwhile, some interesting results were found by study on the SAR. Generally, the methyl group at the 6-position on the indolyl ring was essential for the insecticidal activity. For example, the final mortality rates of 8b-d were 37%, 48.1%, and 44.4%, respectively, while the final mortality rate of 8a, bearing 6-methyl substituent on the indolyl ring, was 55.5%. Interestingly, introduction of the chlorine atom at the 3-position on the benzyl moiety of 8b was important for the insecticidal activity (e.g., 8b versus 8e, 8h, and 8j). The final mortality rates of 8b, 8e (containing meta-Cl), 8h (containing ortho-Cl), and 8j (containing para-Cl) were 37%, 55.5%, 25.9%, and 40.8%, respectively. As described in our previous paper,10 introduction of the substituent on the hydroxyl at the C-4′ position of 5 was necessary for its insecticidal activity (5 versus 8a-g, 8i, and 8j). The above results will encourage us for further structural optimization studies to search for new 4′-substituted indolyl glyoxal derivatives of 4-deoxypodophyllotoxin as the insecticidal agent in future. Finally, the preliminary graphical depiction of the SAR for 5 and 8a-j was summarized in Figure 5.
In conclusion, some novel 4′-substituted indolyl glyoxal derivatives of 4-deoxypodophyllotoxin (8a-j) were semisynthesized from podophyllotoxin, and evaluated for their insecticidal activity against the pre-third-instar larvae of M. separata in vivo. Among all the tested derivatives, compounds 8a, 8e-g, and 8i exhibited more potent insecticidal activity than or comparable to toosendanin at 1 mg/mL. In general, it clearly demonstrated that the methyl group at the 6-position on the indolyl ring, the chlorine atom at the 3-position on the benzyl moiety, and introduction of the substituent on the hydroxyl at the C-4′ position of 4′-demethyl-4-deoxypodophyllotoxin were important for the insecticidal activity.
EXPERIMENTAL
All reagents and solvents were of reagent grade or purified according to standard methods before use. Analytical thin-layer chromatography (TLC) and preparative thin-layer chromatography (PTLC) were performed with silica gel plates using silica gel 60 GF254 (Qingdao Haiyang Chemical Co., Ltd.). Melting points were determined on a digital melting-point apparatus and were uncorrected. Infrared spectra (IR) were recorded on a Bruker TENSOR 27 spectrometer. Proton nuclear magnetic resonance spectra (1H NMR) were recorded on Bruker Avance DMX 400 or 500 MHz instrument, using TMS as the internal standard and CDCl3 as the solvent. Electrospray iontrap mass spectrometry (ESI-MS) was carried out with Thermo Scientific LCQ Fleet mass spectrometer. High-resolution mass spectra (HR-MS) were carried out with APEX II Bruker 4.7T AS instrument.
1-Benzylindoles (6a-j) were synthesized according to the known methods.12,13 Compounds 2 and 5 were prepared starting from compound 1 as described in our previous paper.9
General procedure for the synthesis of O-(deoxypodophyllotoxin-4′-yl)-(N-((un)substituted benzyl)indol-3-yl)glyoxylester derivatives (8a-j)
To a solution of oxalyl chloride (0.65 mmol) in dried dichloromethane (DCM, 5 mL) at 0 °C under N2, a solution of 6a-j (0.5 mmol) in dried DCM (10 mL) was added dropwise. The reaction process was checked by TLC analysis. When the reaction was complete after 2-3 h, the solvent and the excess oxalyl chloride were removed under the reduced pressure to give the intermediates 7a-j, which were used directly for the next step without further purification. To a mixture of 7a-j and Et3N (1 mmol) in dried DCM (10 mL) at 0-5 °C under N2, then a solution of 5 (0.25 mmol) in dried DCM was added dropwise for 1 h. After adding, the mixture was further stirred for 1-2 h, and water (15 mL) was added. Then the organic phase was separated from the water phase, and the latter was extracted with DCM (30 mL × 3). Finally, the combined organic phase was dried over anhydrous Na2SO4, concentrated in vacuo, and purified by preparative thin-layer chromatography (PTLC) to afford the pure products 8a-j, which were well characterized by 1H NMR, MS, HRMS, IR, optical rotation, and mp.
8a: Yield 86%, yellow solid, mp 144-146 oC; [α]20D -23 (C 3.1 mg/mL, CHCl3); IR cm-1: 2921, 2851, 1764, 1640, 1601, 1482, 1380, 1224, 1126, 1037, 929, 698; 1H NMR (400 MHz, CDCl3) δ: 8.43 (s, 1H), 8.35 (d, 1H, J = 8.0 Hz), 7.31-7.33 (m, 3H), 7.16-7.18 (m, 3H), 7.11 (s, 1H), 6.67 (s, 1H, H-5), 6.53 (s, 1H, H-8), 6.41 (s, 2H, H-2′, 6′), 5.93-5.95 (m, 2H, OCH2O), 5.32 (s, 2H, PhCH2), 4.64 (d, J = 3.6 Hz, 1H, H-1), 4.45-4.48 (m, 1H, H-11), 3.90-3.95 (m, 1H, H-11), 3.66 (s, 6H, 3′, 5′-OCH3), 3.06-3.10 (m, 1H, H-4), 2.75-2.81 (m, 3H, H-2, 3, 4), 2.45 (s, 3H, CH3); MS (ESI-TRAP), m/z (%): 660.1 ([M+1]+, 100); HRMS (ESI): Calcd for C39H34NO9 ([M+H]+): 660.2228. Found: 660.2223.
8b: Yield 77%, yellow solid, mp 138-140 oC; [α]20D - 33 (C 3.0 mg/mL, CHCl3); IR cm-1: 2916, 2843, 1765, 1642, 1601, 1225, 1129, 1038, 747, 697; 1H NMR (400 MHz, CDCl3) δ: 8.49-8.53 (m, 2H), 7.28-7.37 (m, 6H), 7.17 (d, J = 6.8 Hz, 2H), 6.66 (s, 1H, H-5), 6.52 (s, 1H, H-8), 6.42 (s, 2H, H-2′, 6′), 5.92-5.96 (m, 2H, OCH2O), 5.36 (s, 2H, PhCH2), 4.65 (d, J = 3.6 Hz, 1H, H-1), 4.46-4.49 (m, 1H, H-11), 3.91-3.96 (m, 1H, H-11), 3.75 (s, 6H, 3′, 5′-OCH3), 3.06-3.11 (m, 1H, H-4), 2.75-2.81 (m, 3H, H-2, 3, 4); MS (ESI-TRAP), m/z (%): 645.93 ([M+1]+, 57); HRMS (ESI): Calcd for C38H32NO9 ([M+H]+): 646.2072. Found: 646.2083.
8c: Yield 68%, yellow solid, mp 136-138 oC; [α]20D - 24 (C 3.3 mg/mL, CHCl3); IR cm-1: 2919, 2850, 1763, 1650, 1601, 1483, 1385, 1225, 1127, 1036, 934, 747, 698; 1H NMR (400 MHz, CDCl3) δ: 8.45 (s, 1H), 7.29-7.34 (m, 3H), 7.08-7.20 (m, 5H), 6.67 (s, 1H, H-5), 6.53 (s, 1H, H-8), 6.42 (s, 2H, H-2′, 6′), 5.94 (d, J = 8.0 Hz, 2H, OCH2O), 5.35 (s, 2H, PhCH2), 4.64 (d, J = 4.0 Hz, 1H, H-1), 4.45-4.49 (m, 1H, H-11), 3.91-3.95 (m, 1H, H-11), 3.67 (s, 6H, 3′, 5′-OCH3), 3.06-3.09 (m, 1H, H-4), 2.95 (s, 3H, CH3), 2.75-2.78 (m, 3H, H-2, 3, 4); MS (ESI-TRAP), m/z (%): 659.79 ([M+1]+, 48); HRMS (ESI): Calcd for C39H34NO9 ([M+H]+): 660.2228. Found: 660.2236.
8d: Yield 60%, yellow solid, mp 136-138 oC; [α]20D - 45 (C 3.1 mg/mL, CHCl3); IR cm-1: 2927, 1739, 1643, 1601, 1483, 1376, 1227, 1128, 1036, 943, 697; 1H NMR (400 MHz, CDCl3) δ: 8.48 (s, 1H), 8.41 (d, 1H, J = 7.6 Hz), 7.27-7.33 (m, 4H), 6.97-7.03 (m, 3H), 6.67 (s, 1H, H-5), 6.53 (s, 1H, H-8), 6.41 (s, 2H, H-2′, 6′), 5.93 (d, J = 8.0 Hz, 2H, OCH2O), 5.62 (s, 2H, PhCH2 ), 4.64 (d, J = 4.0 Hz, 1H, H-1), 4.45-4.49 (m, 1H, H-11), 3.90-3.95 (m, 1H, H-11), 3.66 (s, 6H, 3′, 5′-OCH3), 3.06-3.09 (m, 1H, H-4), 2.74-2.78 (m, 3H, H-2, 3, 4), 2.51 (s, 3H, CH3); MS (ESI-TRAP), m/z (%): 659.83 ([M+1]+, 100); HRMS (ESI): Calcd for C39H34NO9 ([M+H]+): 660.2228. Found: 660.2238.
8e: Yield 55%, yellow solid, mp 128-130 oC; [α]20D - 35 (C 3.1 mg/mL, CHCl3); IR cm-1: IR cm-1: 2915, 2848, 1766, 1642, 1600, 1482, 1390, 1224, 1128, 1037, 911, 748, 680; 1H NMR (500 MHz, CDCl3) δ: 8.53-8.54 (m, 2H), 7.29-7.40 (m, 5H), 7.22 (s, 1H), 7.04 (d, J = 7.0 Hz, 1H), 6.70 (s, 1H, H-5), 6.56 (s, 1H, H-8), 6.45 (s, 2H, H-2′, 6′), 5.94-5.98 (m, 2H, OCH2O), 5.35 (s, 2H, PhCH2), 4.67 (d, J = 4.0 Hz, 1H, H-1), 4.48-4.51 (m, 1H, H-11), 3.93-3.97 (m, 1H, H-11), 3.71 (s, 6H, 3′, 5′-OCH3), 3.09-3.11 (m, 1H, H-4), 2.75-2.80 (m, 3H, H-2, 3, 4); MS (ESI-TRAP), m/z (%): 679.88 ([M+1]+, 80), 681.81 ([M+1]+, 38); HRMS (ESI): Calcd for C38H31NO9Cl ([M+H]+): 680.1682. Found: 680.1671.
8f: Yield 85%, yellow solid, mp 134-136 oC; [α]20D - 41 (C 3.4 mg/mL, CHCl3); IR cm-1: 2917, 2848, 1763, 1641, 1600, 1482, 1380, 1225, 1127, 1039, 930, 773, 680; 1H NMR (400 MHz, CDCl3) δ: 8.44 (s, 1H), 8.35 (d, J = 7.6 Hz, 1H), 7.27-7.29 (m, 2H), 7.18-7.24 (m, 2H), 7.07 (s, 1H), 7.00 (d, J = 6.8 Hz, 1H), 6.67 (s, 1H, H-5), 6.53 (s, 1H, H-8), 6.42 (s, 2H, H-2′, 6′), 5.92-5.96 (m, 2H, OCH2O), 5.30 (s, 2H, PhCH2), 4.65 (d, J = 3.6 Hz, 1H, H-1), 4.45-4.49 (m, 1H, H-11), 3.91-3.95 (m, 1H, H-11), 3.68 (s, 6H, 3′, 5′-OCH3), 3.06-3.11 (m, 1H, H-4), 2.75-2.81 (m, 3H, H-2, 3, 4), 2.45 (s, 3H, CH3); MS (ESI-TRAP), m/z (%): 693.76 ([M+1]+, 29), 695.98 ([M+1]+, 11). HRMS (ESI): Calcd for C39H33NO9Cl ([M+H]+): 694.1838. Found: 694.1843.
8g: Yield 75%, yellow solid, mp 140-142 oC; [α]20D - 48 (C 3.1 mg/mL, CHCl3); IR cm-1: 2921, 2850, 1766, 1644, 1599, 1482, 1384, 1225, 1127, 1036, 943, 750, 680; 1H NMR (400 MHz, CDCl3) δ: 8.46 (s, 1H), 8.42 (d, J = 8.0 Hz, 1H), 7.21-7.27 (m, 3H), 7.03-7.04 (m, 2H), 6.79 (d, J = 7.2 Hz, 1H), 6.67 (s, 1H, H-5), 6.53 (s, 1H, H-8), 6.42 (s, 2H, H-2′, 6′), 5.94 (d, J = 7.2 Hz, 2H, OCH2O), 5.58 (s, 2H, PhCH2), 4.64 (d, J = 3.6 Hz, 1H, H-1), 4.45-4.48 (m, 1H, H-11), 3.90-3.95 (m, 1H, H-11), 3.70 (s, 6H, 3′, 5′-OCH3), 3.06-3.10 (m, 1H, H-4), 2.74-2.81 (m, 3H, H-2, 3, 4), 2.50 (s, 3H, CH3); MS (ESI-TRAP), m/z (%): 693.81 ([M+1]+, 99), 695.82 ([M+1]+, 42). HRMS (ESI): Calcd for C39H33NO9Cl ([M+H]+): 694.1838. Found: 694.1849.
8h: Yield 76%, yellow solid, mp 132-133 oC; [α]20D - 4 (C 3.0 mg/mL, CHCl3); IR cm-1: 2919, 2847, 1766, 1644, 1601, 1482, 1390, 1225, 1128, 1038, 929, 748, 680; 1H NMR (400 MHz, CDCl3) δ: 8.51-8.52 (m, 2H), 7.43 (d, J = 8.4 Hz, 1H), 7.34-7.38 (m, 1H), 7.31-7.32 (m, 2H), 7.24-7.28 (m, 1H), 7.14-7.18 (m, 1H), 6.81 (d, J = 7.6 Hz, 1H), 6.67 (s, 1H, H-5), 6.53 (s, 1H, H-8), 6.42 (s, 2H, H-2′, 6′), 5.94 (d, J = 7.6 Hz, 2H, OCH2O), 5.47 (s, 2H, PhCH2), 4.65 (d, J = 3.6 Hz, 1H, H-1), 4.45-4.49 (m, 1H, H-11), 3.91-3.95 (m, 1H, H-11), 3.70 (s, 6H, 3′, 5′-OCH3), 3.06-3.11 (m, 1H, H-4), 2.75-2.81 (m, 3H, H-2, 3, 4); MS (ESI-TRAP), m/z (%): 679.74 ([M+1]+, 99), 681.90 ([M+1]+, 43). HRMS (ESI): Calcd for C38H31NO9Cl ([M+H]+): 680.1683. Found: 680.1672.
8i: Yield 59%, yellow solid, mp 130-132 oC; [α]20D - 37 (C 3.2 mg/mL, CHCl3); IR cm-1: 2915, 2842, 1765, 1644, 1601, 1482, 1381, 1224, 1127, 1038, 929, 754, 680; 1H NMR (500 MHz, CDCl3) δ: 8.46 (s, 1H), 8.39 (d, J = 8.5 Hz, 1H), 7.45 (d, J = 7.5 Hz, 1H), 7.16-7.22 (m, 3H), 7.11 (s, 1H), 6.80 (d, J = 8.0 Hz, 1H), 6.69 (s,1 H, H-5), 6.55 (s, 1H, H-8), 6.44 (s, 2H, H-2′, 6′), 5.94-5.97 (m, 2H, OCH2O), 5.45 (s, 2H, PhCH2), 4.66 (d, J = 4.0 Hz, 1H, H-1), 4.47-4.50 (m, 1H, H-11), 3.93-3.96 (m, 1H, H-11), 3.70 (s, 6H, 3′, 5′-OCH3), 3.08-3.11 (m, 1H, H-4), 2.75-2.80 (m, 3H, H-2, 3, 4), 2.47 (s, 3H, CH3); MS (ESI-TRAP), m/z (%): 693.83 ([M+1]+, 50), 695.97 ([M+1]+, 21). HRMS (ESI): Calcd for C39H33NO9Cl ([M+H]+): 694.1838. Found: 694.1841.
8j: Yield 65%, yellow solid, mp 132-134 oC; [α]20D - 41 (C 2.9 mg/mL, CHCl3); IR cm-1: 2914, 2846, 1765, 1641, 1600, 1483, 1390, 1225, 1129, 1038, 929, 748, 680; 1H NMR (400 MHz, CDCl3) δ: 8.50-8.51 (m, 2H), 7.28-7.38 (m, 5H), 7.10 (d, J = 8.4 Hz, 2H), 6.68 (s, 1H, H-5), 6.54 (s, 1H, H-8), 6.43 (s, 2 H, H-2′, 6′), 5.94-5.96 (m, 2H, OCH2O), 5.33 (s, 2H, PhCH2), 4.65 (d, J = 4.0 Hz, 1H, H-1), 4.46-4.49 (m, 1H, H-11), 3.91-3.96 (m, 1H, H-11), 3.68 (s, 6H, 3′, 5′-OCH3), 3.06-3.10 (m, 1H, H-4), 2.75-2.82 (m, 3H, H-2, 3, 4); MS (ESI-TRAP), m/z (%): 679.77 ([M+1]+, 100), 681.74 ([M+1]+, 34). HRMS (ESI): Calcd for C38H31NO9Cl ([M+H]+): 680.1682. Found: 680.1755.
Bioassay
The insecticidal activity of 5 and 8a-j against the pre-third-instar larvae of M. separata was assessed by leaf-dipping method as described previously.9 To each compound, 30 larvae (10 larvae per group) were used. Acetone solutions of 5, 8a-j and toosendanin (used as a positive control) were prepared at the concentration of 1 mg/mL. Fresh corn leaves were dipped into the corresponding solution for 3 s, then taken out and dried in a room. Leaves treated with acetone alone were used as a blank control group. Several treated leaves were kept in each dish, where every 10 larvae were raised. If the treated leaves were consumed, the corresponding ones were added to the dish. After 48 h, untreated fresh leaves were added to the all dish until the adult emergence. The experiment was carried out at 25 ± 2 oC and relative humidity (RH) 65-80%, and on 12 h/12 h (light/dark) photoperiod. The insecticidal activity of the tested compounds against the pre-third-instar larvae of M. separata was calculated by the following formula:
Corrected mortality rate (%) = (T−C) ×100/(1−C)
Where T is the mortality rate in the treated group expressed as a percentage, and C is the mortality rate in the untreated group expressed as a percentage.
ACKNOWLEDGEMENTS
This work was financially in part supported by National Natural Science Foundation of China (No. 31071737), the Program for New Century Excellent University Talents, State Education Ministry of China (NCET-06-0868), and the Special Funds of Central Colleges Basic Scientific Research Operating Expenses (QN2009045).
References
1. F. E. Dayan, C. L. Cantrell, and S. O. Duke, Bioorg. Med. Chem., 2009, 17, 4022. CrossRef
2. H. Xu, M. Lv, and X. Tian, Curr. Med. Chem., 2009, 16, 327. CrossRef
3. Y. J. You, Curr. Pharm. Des., 2005, 11, 1695. CrossRef
4. M. Gordaliza, P. A. Garcia, J. M. Miguel del Corral, M. A. Castro, and M. A. Gomez-Zurita, Toxicon, 2004, 44, 441. CrossRef
5. M. Kozawa, K. Baba, Y. Matsuyama, T. Kido, M. Sakai, and T. Takemoto, Chem. Pharm. Bull., 1982, 30, 2885.
6. Y. Inamori, Y. Kato, M. Kubo, K. Baba, Y. Matsuyama, M. Sakai, and M. Kozawa, Chem. Pharm. Bull., 1983, 31, 4464.
7. M. Miyazawa, M. Fukuyama, K. Yoshio, T. Kato, and Y. Ishikawa, J. Agric. Food Chem., 1999, 47, 5108. CrossRef
8. H. Xu, L. Zhang, B. Su, X. Zhang, and X. Tian, Heterocycles, 2009, 77, 293. CrossRef
9. H. Xu, J. J. Wang, H. J. Sun, M. Lv, X. Tian, X. J. Yao, and X. Zhang, J. Agric. Food Chem., 2009, 57, 7919. CrossRef
10. H. Xu and J. J. Wang, Bioorg. Med. Chem. Lett., 2010, 20, 2500. CrossRef
11. G. Bacher, B. Nickel, P. Emig, U. Vanhoefer, S. Seeber, A. Shandra, T. Klenner, and T. Beckers, Cancer Res., 2001, 61, 392.
12. H. Xu and Y. Y. Wang, Chin. J. Chem., 2010, 28, 125. CrossRef
13. H. Heaney and S. V. Ley, Org. Syn., 1974, 54, 58.