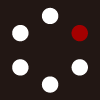
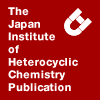
HETEROCYCLES
An International Journal for Reviews and Communications in Heterocyclic ChemistryWeb Edition ISSN: 1881-0942
Published online by The Japan Institute of Heterocyclic Chemistry
e-Journal
Full Text HTML
Received, 17th June, 2011, Accepted, 19th July, 2011, Published online, 21st July, 2011.
DOI: 10.3987/COM-11-S(P)40
■ Reinvestigation of the Classical Total Synthesis of Makomakine
Makoto Furuya, Ayumi Nagatomo, and Masahiro Toyota*
Department of Chemistry, Graduate School of Science, Osaka Prefecture University, 1-1 Gakuencho, Sakai, Osaka 593-8531, Japan
Abstract
Reinvestigation of the original Stevens synthesis of makomakine showed that the yield of the Ritter reaction of 3-indoylacetonitrile with (–)-β-pinene could be increased by employing Hg(OTf)2.Makomakine (1)1 was isolated from Aristotelia serrata in 1981, and it was found that 1 is a biogenetic precursor of aristoteline (2), isolated from A. serrata in 1975 (Figure 1).2
In 1983, Stevens demonstrated an elegant total syntheses of makomakine (1) and aristoteline (2) using the Ritter reaction as a key step of the synthesis.3 The yield of the Ritter reaction in this synthesis was improved from 17% to 39% by Heathcock,4 however, there is room for further yield improvement. Because we had developed a novel methodology for constructing 3-carbomethoxyindole derivatives (Scheme 1),5 we became interested in the syntheses of makomakine (1) and aristoteline (2) and in improving their yields. Herein, we present experimental results related to the Ritter reaction.
Although 3-indolylacetonitrile (5) is commercially available, 4a was converted to 5 in a 96% overall yield5 to enable the analogue syntheses of makomakine (1) and aristoteline (2) using 4b.
We initially examined the Ritter reaction of 5 with (–)-β-pinene using 1.1 equivalents of anhydrous mercuric nitrate according to the literature.4 After the reaction had gone to completion, the resulting crude product was subjected to NaBH4 reduction to provide makomakine (1) in a 41% yield (entry 1). Performance of the Ritter reaction and the subsequent hydride reduction in the presence of catalytic amounts of anhydrous mercuric nitrate provided a trace amount of 1, as detected by 1H NMR spectroscopy (entry 2). To improve the environmental impact of the synthesis, the Ritter reaction was performed in the presence of a variety of harmless metals. Disappointingly, CuOTf, AuCl, Pd(OAc)2, PdCl2, and Pd(OCOCF3)2 did not yield the desired products (entries 3–7). Fortunately, the yield of the Ritter reaction was increased by employing Hg(OTf)2, and makomakine (1) was obtained in a 72% overall yield in two steps (entry 8).6 It should be noted that the Ritter reaction using catalytic amounts of Hg(OTf)2 met with failure. Finally, makomakine (1) was transformed into aristoteline (2) in 60% yield by applying Stevens’ protocol.3
In conclusion, Hg(OTf)2 proved to be an efficient reagent for the Ritter reaction of 3-indolylacetonitrile with (–)-β-pinene in the makomakine synthesis. The methodology developed here should also provide access to synthetic analogues of makomakine (1) and aristoteline (2).
EXPERIMENTAL
IR spectra were measured on a SHIMADZU FT-IR 8300 spectrophotometer. 1H NMR spectra were recorded on Varian 400 MR (400 MHz) spectrometers with CHCl3 (δ 7.26) as an internal standard. 13C NMR spectra were recorded on JEOL JX-500 (125 MHz) spectrometer with CHCl3 (δ 77.16) as an internal standard. All compounds purified by chromatography were sufficiently pure (> 95% by 1H NMR analysis) for use in subsequent reactions.
(+)-Makomakine (1). To a stirred solution of 3-indoylacetonitrile (5) (1.87 g, 12.0 mmol) and Hg(OTf)2 (997.46 mg, 2.0 mmol) in dry CH2Cl2 (10 mL) was added dropwise (−)-β-pinene (0.28 mL, 1.8 mmol) at −40 °C, and then the resulting mixture was allowed to warm to room temperature over 3 h. After cooling to 0 °C, 3 M NaOH in MeOH (7.2 mL) was added. Slow addition of an excess amount of NaBH4 and stirring at 0 °C gave the mixture which was filtered through Celite. The organic layer was separated and the aqueous layer was extracted with EtOAc. The combined organic layers were washed with brine, dried, and evaporated to afford the crude product which was purified by flash column chromatography. Elution with hexane-acetone (12:1) and 3% triethylamine afforded makomakine (1) (380.8 mg, 72% for 2 steps) as a solid. [α] D25 +112.2 (c 0.80, CHCl3) (lit.1 [α]D19 +131.2 (c 0.5, CHCl3), Mp 95-97 °C (lit.1 99-100 °C). 1H NMR (CDCl3, 400 MHz): δ 1.10 (s, 3H), 1.14 (s, 3H), 1.38-1.42 (m, 1H), 1.49 (dddd, 1H, J = 4.0, 6.0, 13.6 and 13.6 Hz), 1.58 (ddd, 1H, J = 3.6, 3.6 and 12.8 Hz), 2.04-2.11 (m, 1H), 2.12-2.15 (m, 1H), 2.14-2.22 (m, 1H), 2.25-2.29 (m, 1H), 2.62 (dd, 1H, J = 8.0 and 14.4 Hz), 2.76 (dd, 1H, J = 6.0 and 14.4 Hz), 3.02-3.12 (m, 1H), 3.49 (ddd, 1H, J = 2.8, 6.0 and 8.0 Hz), 4.58 (dd, 1H, J = 2.4 and 2.4 Hz), 4.77 (dd, 1H, J = 2.4 and 2.4 Hz), 7.02 (d, 1H, J = 2.0 Hz), 7.10 (ddd, 1H, J = 0.8, 7.2 and 7.2 Hz), 7.10 (ddd, 1H, J = 0.8, 7.2 and 7.2 Hz), 7.19 (ddd, 1H, J = 0.8, 7.2 and 7.2 Hz), 7.36 (ddd, 1H, J = 0.8, 0.8 and 8.4 Hz), 7.64 (dd, 1H, J = 0.8 and 8.4 Hz), and 7.95 (br s, 1H). 13C NMR (CDCl3, 125 MHz): δ 150.6, 136.5, 128.0, 122.5, 121.9, 119.3, 119.1, 113.8, 111.1, 108.9, 54.2, 53.2, 43.4, 36.8, 33.3, 32.1, 31.5, 29.9, 29.4, and 27.2. IR (CHCl3, cm–1) 3416, 3061, 2923, 1637, 1455, 886, and 740. LRMS m/z 294 (M+). HRMS calcd for C20H26N2 294.2096, found: 294.2099.
References
1. I. R. C. Bick and M. A. Hai, Heterocycles, 1981, 16, 1301. CrossRef
2. B. F. Anderson, G. B. Robertson, H. P. Avey, W. F. Donovan, I. R. C. Bick, J. B. Bremner, A. J. T. Finney, N. W. Preston, R. T. Gallagher, and G. B. Russell, J. Chem. Soc., Chem. Commun., 1975, 511. CrossRef
3. R. V. Stevens and P. M. Kenney, J. Chem. Soc., Chem. Commun., 1983, 384. CrossRef
4. D. Stoermer and C. H. Heathcock, J. Org. Chem., 1993, 58, 564; CrossRef Chemistries and Syntheses related to makomakine (1) and aristoteline (2): J. E. Saxton, ‘Monoterpenoid Indole Alkaloids,’ Vol. 25, Wiley, Chichester, 1994, Supplement to Part 4, pp. 15-34.
5. (a) M. Toyota and K. Fukumoto, J. Chem. Soc., Perkin Trans. 1, 1992, 547; CrossRef (b) M. Toyota and F. Fukumoto, Heterocycles, 1990, 31, 1431. CrossRef
6. M. Nishizawa, E. Morikuni, K. Asoh, Y. Kan, K. Uenoyama, and H. Imagawa, Synlett, 1995, 169. CrossRef
7. T. Darbre, C. Nussbaumer, and H. J. Borschberg, Helv. Chim. Acta, 1984, 67, 1040. CrossRef