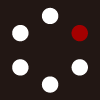
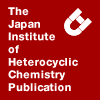
HETEROCYCLES
An International Journal for Reviews and Communications in Heterocyclic ChemistryWeb Edition ISSN: 1881-0942
Published online by The Japan Institute of Heterocyclic Chemistry
e-Journal
Full Text HTML
Received, 11th October, 2012, Accepted, 15th November, 2012, Published online, 28th November, 2012.
■ Application of Zirconacyclopentadienes (Metalla-heterocycles) and Cross-Coupling for the Convenient Preparative Method of 6,13-Disubstituted Pentacene
Zhiying Jia, Shi Li, Kiyohiko Nakajima, Ken-ichiro Kanno, Zhiyi Song, and Tamotsu Takahashi*
Catalysis Research Center and Graduate School of Pharmaceutical Science, Hokkaido University, Sapporo 001-0021, Japan
Abstract
Iodination of zirconacyclopentadiene derivative gave diiododiene derivative. The product was lithiated with t-BuLi and treated with diiodonaphthalene successively to afford 6,13-bis(trimethylsilyl)-5,14-dihydropentacene. A 6,13-diiodo-5,14-dihydropentacene was synthesized by iodination of 6,13-bis(trimethylsilyl)-5,14-dihydropentacene with ICl. This diiododihydropentacene was used for the introduction of substituent at 6 and 13 positions by the cross-coupling reactions with Pd catalyst. After aromatization by a combination of DDQ and γ-terpinene or triethylamine, 6,13-disubstituted pentacene derivatives were synthesized.1. INTRODUCTION
Zirconacyclopentadienes are versatile metalla-heterocyclic compounds for aromatic compounds formation such as benzene derivatives,1 pyridine derivatives,2 and multi-aromatic compounds.3 Zirconacyclopentadienes are conveniently prepared from Cp2ZrBu2 (Negishi reagent) and two alkynes or diynes.4 We have reported coupling reaction of zirconacyclopentadienes with diiodobenzene or tetraiodobenzene in the presence of CuCl for extension of aromatic rings as shown in eq. 1.5 However, trimethylsilyl-substituted zirconacyclopentadienes did not react with diiodobenzene even in the presence of CuCl.
In order to circumvent this difficulty, we developed an alternative route for the coupling reaction of trimethylsilyl-substituted zirconacyclopentadienes with diiodobenzene. We have preliminarily reported the alternative method which involves diiodination, lithiation and coupling with diiodobenzene in the presence of CuCl (eq. 2).6 In this paper, we would like to report the combination of this alternative method and cross-coupling reaction for the application to a convenient preparative method of 6,13-disubstituted pentacene derivatives.
Pentacene derivatives, are known for good p-type semiconductor because of the high charge carrier mobility, have been extensively studied as organic materials7 and in particular, 6,13-disubstituted pentacene derivatives have been prepared by the quinone method (eq. 3).8 The reaction of Grignard reagents with pentacene quinone sometimes did not occur at the carbonyl moiety but at the next ring like Michael addition to α,β-unsaturated ketone moiety to give undesired products.9 In the case of addition of thienyl groups, it was observed that the second thienyl group migrated to the unexpected position to afford the by-products.10
This situation prompted us to develop novel method to prepare 6,13-disubstituted pentacene. For this purpose, we combine the coupling reaction of silyl-substituted tricyclic zirconacyclopentadienes with diiodonaphthalene giving disilyldihydropentacene, and introduction of substituents by cross-coupling reaction such as Negishi coupling, Suzuki-Miyaura coupling and so on, after iodination of trimethylsilyl substituents of disilyldihydropentacene. These reactions are shown in Scheme 1.
2. RESULTS AND DISCUSSION
2.1. Synthesis of a 6,13-diiodo-5,14-dihydropentacene
We have recently reported the formation of 6,13-bis(trimethylsilyl)-5,14-dihydropentacene 5 by Zr-mediated method.11 It is worth noting that two trimethylsilyl groups of which are capable of further functionalizing. Encouraged by this, we synthesized a 6,13-diiodo-5,14-dihydropentacene 6 from compound 5 by a simple iodination with 2.2 equiv of iodine monochloride (Scheme 2).
This iodo compound could be converted to a variety of pentacene derivatives basically via two steps: coupling and aromatization.
Recently Chi and co-workers prepared multi-substituted dibromopentacene derivatives from hexabromide.12
2.2. Coupling reactions of 6,13-diiodo-5,14-dihydropentacene
There are two choices due to the order of two steps, coupling with metal reagents followed by aromatization or aromatization followed by coupling. Since a diiodopentacene, which should be formed by doing the aromatization first, was anticipated with poor solubility and poor stability, subsequently, the coupling reactions of compound 6 were firstly carried out. Alkyl, aryl, alkynyl, and alkenyl groups were introduced by cross-coupling reactions in the presence of Pd catalyst (Scheme 3).
2.2.1 Negishi Cross-Coupling Reaction
6,13-Diiodo-5,14-dihydropentacene 6 was treated with three equiv. of trimethylaluminium or triethylaluminium in the presence of 5mol% PdCl2(PPh3)2 catalyst in THF at reflux for 24 h to give products 7a or 7b in 86 or 84% yield, respectively (Scheme 4). When compound 6 reacted with Zinc reagents in the presence of Pd(PPh3)4 catalyst, 6,13-phenyl-, thienyl-, bis-thienyl-substituted 5,14-dihydropentacenes 7c-e were synthesized successfully by the typical Negishi coupling reaction.
2.2.2 Sonogashira Cross-Coupling Reaction
Sonogashira coupling reaction was carried out to form alkynyl group substituted products (Scheme 5). Five equiv. of trimethylsilylacetylene or triisopropylsilylacetylene were empolyed in these reactions to ensure that all the starting compound 6 was consumed. Corrossponding producs 7f and 7g were produced in high yields of 92 and 96%, respectively. The isolated yields were 84 and 87%.
2.2.2 Suzuki Cross-Coupling Reaction
Alkene substituted dihydropentacene could also be obtained by the coupling reaction of the diiodo compound 6. One example was examined in this issue as shown in Scheme 6.
Suzuki-Miyaura coupling was applied to synthesize 6,13-dialkenyl-5,14-dihydropentacene 7h in 92% NMR yield. Isolated yield of this case was only 54%, which is possibly due to its polymerization on the silica column.
2.3. Formation of 6,13-disubstituted pentacenes
As we planned, thus obtained 6,13-disubstituted-5,14-dihydropentacenes 7 were aromatized by DDQ and 50 equiv. of γ-terpinene or triethylamine at 80 °C for 3 h to give 6,13-disubstituted pentacenes 8. The results are shown in Scheme 7. Dimethylpentacene and diethylpentacene could be prepared in 45 and 42% yields, respectively. Both of them were isolated and characterized by NMR successfully. Such alkyl substituted pentacene derivatives are very rear.13 Aryl substituted pentacenes, such as phenyl, thienyl or bis-thienyl, were also synthesized in 72, 67 and 70% yields, respectively. Moveover, alkynyl-substituted pentacenes were prepared in high yields of 81 and 90%, respectively. The aromatization of dialkenyl dihydropentacene 7h was failed under our typical conditions.
3. CONCLUSIONS
In summary, 6,13-diiodo-5,14-dihydropentacene was synthesized from a 6,13-bis(trimethylsilyl)-5,14- dihydropentacene. Introduction of alkyl, aryl, alkynyl, alkenyl groups was carried out by the palladium catalyzed cross-coupling reaction. One additional step of aromatization gave the 6,13-disubstituted pentacene derivatives in good yields.
4. EXPERIMENTAL
All reactions involving air- or moisture-sensitive organometallic reagents were carried out under dry nitrogen. THF, toluene, pentane, and diethyl ether were distilled over sodium and benzophenone. 1,3-Dimethyl-3,4,5,6-tetrahydro-2(1H)-pyrimidinone (DMPU; Aldrich, 98%) was dried over calcium hydride and distilled under reduced pressure. Zirconocene dichloride (Tokyo Chemical Industries, Ltd.), copper(I) chloride (Wako Pure Chemical Industries, Ltd., 99.9%), 2,3-dichloro-5,6-dicyano-1,4- benzoquinone (DDQ, Kanto, 98%), γ-terpinene (Wako Pure Chemical Industries, Ltd.), and trans-1-penten-1-ylboronic acid pinacol ester (Aldrich, 97%) were used as received. Zinc dichloride was dried by heat gun in vacuo. 6,13-Bis(trimethylsilyl)-5,14-dihydropentacene was synthesized by our reported method.11 PdCl2(PPh3)2 is commercially available catalyst. Pd(PPh3)4 was prepared according to literature.14 1H and 13C NMR spectra were recorded in CDCl3 or C6D6 (containing 1% TMS) solutions on a JEOL JNM-ECX400 or a JEOL JNM-ECA600 spectrometer.
4.1. Preparation of 6,13-diiodo-5,14-dihydropentacene 6
To a THF solution (5 mL) of 6,13-bis(trimethylsilyl)-5,14-dihydropentacene (424 mg, 1.0 mmol) was slowly added ICl (1.0M CH2Cl2 solution, 2.2 mL, 2.2 mmol) at -78 °C. After stirring at this temperature for 3 h, the reaction mixture was quenched with saturated aqueous Na2S2O3, and extracted with CHCl3. The combined organic phase was washed with brine. After removal of the solvent, CHCl3 was added again to appear large amount of precipitate. After filtration, filter cake was collected as a brown solid (255 mg, 48% yield).
6: 1H NMR (CDCl3, Me4Si) δ 4.47 (s, 4 H), 7.25-7.29 (m, 2 H), 7.43-7.44 (m, 2 H), 7.52-7.55 (m, 2 H), 8.09-8.12 (m, 2 H), 8.85 (s, 2 H); 13C NMR (CDCl3, Me4Si) δ 45.5, 106.7, 126.5, 126.9, 127.2, 128.1, 132.1, 132.8, 133.0, 136.3, 139.8. HRMS (EI) calcd for C22H14I2: 531.9185. Found: 531.9199.
4.2. Coupling reactions of 6,13-diiodo-5,14-dihydropentacene 6
4.2.1. Preparation of 6,13-dimethyl-5,14-dihydropentacene 7a and 6,13-diethyl-5,14-dihydro- pentacene 7b
To a solution of 6,13-diiodo-5,14-dihydropentacene 2 (161 mg, 0.30 mmol) and PdCl2(PPh3)2 (10 mg, 0.015 mmol) in THF (10 mL) was added AlMe3 (1.0 M hexane solution, 0.9 mL, 0.9 mmol), and the mixture was heated to reflux for 1 d. After cooling to room temperature, the mixture was quenched with 3 M aqueous HCl and extracted with CHCl3. Removal of the solvent, the residue was purified by column chromatography (hexane : EtOAc = 10 : 1) to afford the title compound 7a (76 mg, 82% isolated yield) as pale yellow solid.
7a: pale yellow solid. 1H NMR (CDCl3, Me4Si, 600 MHz) δ 2.78 (s, 6 H), 4.10 (s, 4 H), 7.14-7.16 (m, 2 H), 7.28-7.30 (m, 2 H), 7.34-7.36 (m, 2 H), 7.92-7.93 (m, 2 H), 8.50 (s, 2 H); 13C NMR (CDCl3, Me4Si) δ 15.1, 34.4, 123.0, 125.1, 126.4, 127.1, 127.3, 128.3, 130.8, 131.0, 132.6, 137.3. HRMS (EI) calcd for C24H20: 308.1565. Found: 308.1565.
Compound 7b was prepared by the same way as described for 7a in 75% yield.
7b: yellow solid. 1H NMR (CDCl3, Me4Si, 600 MHz) δ 1.40 (t, J = 7.1 Hz, 6 H), 3.38 (q, J = 7.1 Hz, 4 H), 4.15 (s, 4 H), 7.22-7.23 (m, 2 H), 7.37-7.38 (m, 2 H), 7.41-7.43 (m, 2 H), 8.00-8.01 (m, 2 H), 8.62 (s, 2 H); 13C NMR (CDCl3, Me4Si) δ 15.0, 22.0, 34.0, 122.9, 125.0, 126.5, 127.0, 128.4, 129.9, 131.0, 132.4, 133.4, 137.9. HRMS (EI) calcd for C26H24: 336.1878. Found: 336.1868.
4.2.2. Preparation of 6,13-diaryl-5,14-dihydropentacene 7c-e
General procedure:
In a Schlenk tube, the arylzinc reagent was prepared in situ by reaction of aryllithium (0.14 mL, 0.27 mmol) with dried ZnCl2 (52 mg, 0.4 mmol) in THF (2 mL). To which 6,13-diiodo-5,14-dihydropentacene 6 (50 mg, 0.09 mmol) was added, and the reaction mixture was degassed by freeze-pump-thaw cycle for three times. Then a Pd(PPh3)4 catalyst (5.2 mg, 0.0045 mmol) was added and the mixture was heated at 50 °C for 12 h. After cooling to room temperature, the reaction mixture was quenched with 3 N HCl and extracted with CHCl3. The combined organic phase was washed with water, saturated aqueous NaHCO3, and brine in successive. The solution was dried over anhydrous MgSO4, filtered, and distilled at reduced pressure to remove the solvent. The residual solid was purified by a silica gel column chromatography or GPC to afford the title compound.
7c: 70% isolated yield, 74% NMR yield, yellow solid. 1H NMR (CDCl3, Me4Si, 400 MHz) ) δ 3.87 (s, 4 H), 7.14 (s, 4 H), 7.30-7.33 (m, 2 H), 7.44-7.46 (m, 4 H), 7.56-7.66 (m, 6 H), 7.75-7.77 (m, 2 H), 7.97 (s, 2 H); 13C NMR (CDCl3, Me4Si) δ 35.5, 125.2, 125.3, 126.4, 127.1, 127.5, 128.3, 128.8 (two peaks were overlapped), 130.7, 130.8 (two peaks were overlapped), 131.0, 133.1, 136.0, 137.7, 139.8. HRMS (EI) calcd for C34H24: 432.1878. Found: 432.1881.
7d: 72% isolated yield, 94% NMR yield, yellow solid. 1H NMR (CDCl3, Me4Si) δ 4.00 (s, 4 H), 7.18-7.25 (m, 6 H), 7.37-7.40 (m, 4 H), 7.65-7.66 (m, 2 H), 7.85-7.87 (m, 2 H), 8.19 (s, 2 H); 13C NMR (CDCl3, Me4Si) δ 35.6, 125.1, 125.5, 126.5, 126.6, 127.2, 127.5, 128.4, 128.6, 129.4, 131.38, 131.43, 135.9, 137.3, 139.7. HRMS (EI) calcd for C30H20S2: 444.1006. Found: 444.1003.
7e: 70% isolated yield, 86% NMR yield, yellow solid. 1H NMR (CDCl3, Me4Si) δ 4.08 (s, 4 H), 7.07 (d, J = 2.4 Hz, 2 H), 7.08-7.10 (m, 2 H), 7.17-7.19 (m, 2 H), 7.24-7.25 (m, 2 H), 7.29 (d, J = 3.6 Hz, 2 H), 7.31 (d, J = 2.4 Hz, 2 H), 7.38-7.39 (m, 2 H), 7.42 (d, J = 2.4 Hz, 2 H), 7.87-7.89 (m, 2 H), 8.32 (s, 2 H); 13C NMR (CDCl3, Me4Si) δ 35.7, 124.1, 124.2, 124.7, 125.2, 125.7, 126.7, 127.3, 128.1, 128.5, 129.3, 129.6, 131.4, 131.8, 136.1, 137.2, 137.7, 138.9, 139.0. HRMS (EI) calcd for C38H24S4: 608.0761. Found: 608.0759.
4.2.3. Preparation of 6,13-bis(trimethylsilylethynyl)-5,14-dihydropentacene 7f and 6,13- bis(triisopropylsilylethynyl)-5,14-dihydropentacene 7g
A 20-mL Schlenk flask was charged with trimethylsilylacetylene (70 uL, 0.5 mmol), compound 6 (53 mg, 0.1 mmol), PdCl2(PPh3)2 (3.51 mg, 0.005 mmol), and CuI (1 mg, 0.005 mmol) under a steam of nitrogen. Freshly distilled triethylamine (3 mL) was added into the flask via syringe, and the reaction mixture was degassed by freeze-pump-thaw cycle for three times. Then the reaction mixture was stirred at 50 °C for 6 h. The solvent was evaporated in vacuo, the resulting residue was dissolved in CHCl3 (25 mL) then washed with saturated aqueous NaHCO3 and brine then dried over anhydrous Na2SO4. After removal of solvent, the residue was purified by silica gel chromatography (hexane : EtOAc = 50 : 1) to afford the title compound 3f (40 mg, 84% isolated yield) as a yellow solid.
7f: 1H NMR (CDCl3, Me4Si) δ 0.48 (s, 18 H), 4.40 (s, 4 H), 7.26-7.28 (m, 2 H), 7.41-7.42 (m, 2 H), 7.50-7.51 (m, 2 H), 8.07-8.08 (m, 2 H), 8.90 (s, 2 H); 13C NMR (CDCl3, Me4Si) δ 0.4, 35.8, 102.0, 106.7, 118.4, 125.4, 125.9, 126.6, 127.7, 128.6, 129.8, 132.1, 136.1, 139.0. HRMS (EI) calcd for C32H32Si2: 472.2043. Found: 472.2045.
The title compound 3g (56 mg) was prepared by the same way as described for 3f in 87% yield from 2 (53 mg, 0.1 mmol).
7g: 1H NMR (CDCl3, Me4Si) δ 1.31 (s, 6 H), 1.32 (s, 36 H), 4.46 (s, 4 H), 7.24-7.27 (m, 2 H), 7.34-7.36 (m, 2 H), 7.48-7.51 (m, 2 H), 7.99-8.02 (m, 2 H), 8.99 (s, 2 H); 13C NMR (CDCl3, Me4Si) δ 11.5, 18.9, 35.8, 103.0, 103.6, 118.4, 125.4, 125.7, 126.5, 127.5, 128.4, 129.9, 131.9, 136.1, 138.8. HRMS (EI) calcd for C44H56Si2: 640.3921. Found: 640.3920.
4.2.4. Preparation of 6,13-di-pent-1-enyl-5,14-dihydropentacene 7h
The trans-1-penten-1-ylboronic acid pinacol ester (170 mg, 1.5 mmol), compound 6 (266 mg, 0.5 mmol) were added to a 50 mL Schlenk flask in THF (10 mL). Aqueous NaOH solution (2 M, 1 mL) was added under N2, and the reaction mixture was degassed by freeze-pump-thaw cycle for three times. Then Pd(PPh3)4 (17 mg, 0.015 mmol) was added, and the reaction was heated under reflux. The reaction was mornitored by NMR and worked up after 1 d. The cooled mixture was extracted with CHCl3, washed with brine and then dried over Na2SO4. The crude product was purified by silica gel chromatography (hexane) to afford the title compound 7h (152 mg, 54% isolated yield) as a yellow solid.
7h: 1H NMR (C6D6, Me4Si) δ 1.17 (t, J = 7 Hz, 6 H), 1.70-1.79 (m, 4 H), 2.49-2.55 (m, 4 H), 4.23 (s, 4 H), 5.94 (dt, J = 16 Hz, J = 6.8 Hz, 2 H), 6.92 (d, J = 16 Hz, 2 H), 7.21-7.23 (m, 2 H), 7.33-7.35 (m, 2 H), 7.42-7.44 (m, 2 H), 7.97-8.00 (m, 2 H), 8.61 (s, 2 H); 13C NMR (CDCl3, Me4Si) δ14.1, 22.9, 35.4, 35.8, 124.4, 125.0, 126.3, 126.7, 127.1, 128.4, 130.1, 131.0, 131.9, 132.7, 138.1, 138.4. HRMS (EI) calcd for C32H32: 416.25040. Found: 416.24912.
4.3. Synthesis of 6,13-disubstituted pentacenes via aromatization
4.3.1. Synthesis of 6,13-dimethylpentacene 8a via a 6,13-dimethylpentacene-DDQ adduct 7aa
In a 20 mL Schlenk tube, 7a (30 mg, 0.1 mmol) and DDQ (45 mg, 0.2 mmol) were mixed in toluene (2 mL). This reaction mixture was degassed and stirred at room temperature for 2 h. The end of reaction was detected by TLC. The solvent was removed by a rotary evaporator, and the residue was purified by silica gel column chromatography (hexane: EtOAc = 2:1) to afford the corresponding adduct 7aa (30 mg, 48% isolated yield and 86% NMR yield) as a red solid (Scheme 8).
DDQ adduct 7aa (533 mg, 1.0 mmol) was mixed with triethylamine (3 mL) in toluene (3 mL). The mixture was degassed and heated to 80 °C for 3 h. After cooling to room temperature, the solvent was removed in vacuo. The residue was washed by MeOH (degassed, 15 mL) to afford the title compound 8a (184 mg, 45% yield) as a blue solid.
7aa: yellow solid. 1H NMR (CDCl3, Me4Si, 400 MHz) δ 2.39 (s, 6 H), 7.57-7.59 (m, 4 H), 7.76 (s, 2 H), 7.82-7.84 (m, 2 H), 7.92-7.94 (m, 2 H), 8.07 (s, 2 H); 13C NMR (CDCl3, Me4Si) δ 15.5, 50.6, 63.4, 113.7, 123.9, 124.0, 127.6, 128.2 (two peaks were overlapped), 128.5, 132.8, 132.9, 134.5, 135.2, 142.3, 178.5. HRMS (FAB) calcd for C32H18O2N2Cl2Na (M+Na+): 555.0643. Found: 555.0618.
8a: blue solid. 1H NMR (C6D6, Me4Si) δ 3.12 (s, 6 H), 7.18-7.20 (m, 4 H), 7.84-7.87 (m, 4 H), 8.86 (s, 4 H); 13C NMR (CDCl3, Me4Si) δ 15.0, 124.4, 125.4, 128.6, 129.0, 129.2, 131.5. UV-vis (CHCl3) λmax/nm: 604, 555, 523. HRMS (EI) calcd for C24H18: 306.14085. Found: 306.13859.
4.3.2. Preparation of 6,13-diethylpentacene 8b
To a degassed toluene solution (3 mL) of 7b (88 mg, 0.2 mmol) was added DDQ (90 mg, 0.4 mmol). The reaction mixture was stirred at room temperature for 5 h. Corresponding DDQ adduct was formed in around 93% NMR yield. This reaction mixture was added with degassed γ-terpinene (1.6 mL, 10 mmol) and heated at 80 °C for 6 h. Again the reaction mixture was cooled to room temperature. The solvent was removed under reduced pressure to leave a solid, which was washed with degassed MeOH (15 mL) for 3 times. The solid was then recrystallized from hexane (degassed) to afford the title compound 8b (28 mg, 0.084 mmol) as blue crystals in 42% yield.
8b: 1H NMR (C6D6, Me4Si) δ 1.50 (t, J = 7.0 Hz, 6 H), 3.83 (q, J = 7.1 Hz, 4 H), 7.14-7.16 (m, 4 H), 7.81-7.83 (m, 4 H), 8.92 (s, 4 H); 13C NMR (C6D6, Me4Si) δ 15.8, 22.4, 124.0, 125.4, 128.4, 129.1, 131.8, 135.5. UV-vis (CHCl3) λmax/nm: 604, 557, 518. HRMS (EI) calcd for C26H22: 334.1721. Found: 334.1712.
4.3.3. General procedure for preparation of 6,13-diphenylpentacene 8c-g
To a degassed toluene solution (3 mL) of dihydropentacene derivative 7 (0.1 mmol) was added DDQ (0.2 mmol). The reaction mixture was stirred at room temperature for 1 h and heated to 50 °C for 3 h. The corresponding DDQ adducts were formed in high yields. It was then treated with γ-terpinene (0.8 mL, 5 mmol) at 80°C for 6 h before remove the solvent, the remained solid was washed by degassed MeOH (15 mL) for 3 times and analyzed by 1H NMR spectroscopy to give NMR yield of 8. Compounds 8c,15 8d,15 8e,16 8f,17 8g18 were identified according to data reported in references.
References
1. T. Takahashi, M. Kotora, and Z. Xi, J. Chem. Soc., Chem. Comm., 1995, 361; CrossRef T. Takahashi, Z. Xi, A. Yamazaki, Y. Liu, K. Nakajima, and M. Kotora, J. Am. Chem. Soc., 1998, 120, 1672; CrossRef L. Zhou, S. Li, K. Kanno, and T. Takahashi, Heterocycles, 2010, 80, 725; CrossRef T. Takahashi, F.-Y. Tsai, Y. Li, K. Nakajima, and M. Kotora, J. Am. Chem. Soc., 1999, 121, 11093; CrossRef T. Takahashi, F.-Y. Tsai, and Y. Li, Chem. Lett., 1999, 11, 1173; CrossRef T. Takahashi, M. Ishikawa, and S. Huo, J. Am. Chem. Soc., 2002, 124, 388; CrossRef Y. R. Dumond and E. Negishi, Tetrahedron, 2004, 60, 1345; CrossRef D. Payra, Z. Song, Kanno, K. Kanno, and T. Takahashi, Chem. Lett., 2011, 40, 1447; CrossRef C. Chen, X. Yan, and C. Xi, Synth. Commun., 2010, 40, 570; CrossRef S. Li, H. Qu, L. Zhou, K. Kanno, Q. Guo, B. Shen, and T. Takahashi, Org. Lett., 2009, 11, 3318; CrossRef J. Liu, W. Zhang, X. Guo, Z. Hou, and Z. Xi, Organometallics, 2007, 26, 6812; CrossRef T. Yu, X. Sun, C. Wang, L. Deng, and Z. Xi, Chem. Eur. J., 2005, 11, 1895; CrossRef L. Dufkova, M. Kotora, and I. Cisarova, Eur. J. Org. Chem., 2005, 12, 2491; CrossRef T. Seki, Y. Noguchi, Z. Duan, W. Sun, and T. Takahashi, Tetrahedron Lett., 2004, 45, 9041. CrossRef
2. T. Takahashi, F.-Y. Tsai, and K. Martin, J. Am. Chem. Soc., 2000, 122, 4994; CrossRef L. Zhou, M. Yamanaka, K. Kanno, and T. Takahashi, Heterocycles, 2008, 76, 923. CrossRef
3. T. Takahashi, M. Kitamura, B. Shen, and K. Nakajima, J. Am. Chem. Soc., 2000, 122, 12876; CrossRef T. Takahashi, S. Li, W. Huang, F. Kong, K. Nakajima, B. Shen, T. Ohe, and K. Kanno, J. Org. Chem., 2006, 71, 7967; CrossRef S. Li, L. Zhou, Z. Song, F. Bao, K. Kanno, and T. Takahashi, Heterocycles, 2007, 73, 519; CrossRef S. Li, Z. Li, K. Nakajima, K. Kanno, and T. Takahashi, Chem. Asian J., 2009, 4, 294; CrossRef T. Takahashi, Y. Li, J. Hu, F. Kong, K. Nakajima, L. Zhou, and K. Kanno, Tetrahedron Lett., 2007, 48, 6726; CrossRef M. T. Stone and H. L. Anderson, J. Org. Chem., 2007, 72, 9776. CrossRef
4. E. Negishi, F. E. Cederbaum, and T. Takahashi, Tetrahedron Lett., 1986, 27, 2829; CrossRef E. Negishi and T. Takahashi, Acc. Chem. Res., 1994, 27, 124. CrossRef
5. T. Takahashi, R. Hara, Y. Nishihara, and M. Kotora, J. Am. Chem. Soc., 1996, 118, 5154; CrossRef T. Takahashi,Y. Li, P. Stepnicka, M. Kitamura, Y. Liu, K. Nakajima, and M. Kotora, J. Am. Chem. Soc., 2002, 124, 576; CrossRef X. Zhou, Z. Li, H. Wang, M. Kitamura, K. Kanno, K. Nakajima, and T. Takahashi, J. Org. Chem., 2004, 69, 4559; CrossRef T. Takahashi, K. Kashima, S. Li, K. Nakajima, and K. Kanno, J. Am. Chem. Soc., 2007, 129, 15752; CrossRef Y. Ni, K. Nakajima, K. Kanno, and T. Takahashi, Org. Lett., 2009, 11, 3702; CrossRef T. Seri, H. Qu, L. Zhou, K. Kanno, and T. Takahashi, Chem. Asian J., 2008, 3, 388; CrossRef W. Huang, X. Zhou, K. Kanno, and T. Takahashi, Org. Lett., 2004, 6, 2429. CrossRef
6. L. Zhou, K. Nakajima, K. Kanno and T. Takahashi, Tetrahedron Lett., 2009, 50, 2722. CrossRef
7. D. J. Gundlach, Y.-Y. Lin, T. N. Jackson, D. F. Nelson, S. F. Nelson, and D. G. Schlom, IEEE Electron Device Lett., 1997, 18, 87; CrossRef Y.-Y. Lin, D. J. Gundlach, D. F. Nelson, and T. N. Jackson, IEEE Electron Device Lett., 1997, 18, 606; CrossRef Y.-Y. Lin, D. J. Gundlach, and T. N. Jackson, Appl. Phys. Lett., 1998, 72, 1854; CrossRef V. Coropceanu, J. Cornil, D. A. Da Silva Filho, Y. Olivier, R. Silbey, and J. Bredas, Chem. Rev., 2007, 107, 926; CrossRef V. C. Sundar, J. Zaumseil, V. Podzorov, E. Menard, R. L. Willet, T. Someya, M. E. Gershenson, and J. A. Rogers, Science, 2004, 303, 1644. CrossRef
8. S. Li, L. Zhou, K. Nakajima, K. Kanno, and T. Takahashi, Chem. Asian J., 2010, 5, 1620; CrossRef D. R. Maulding and B. G. Roberts, J. Org. Chem., 1969, 34, 1734. CrossRef
9. C. F. H. Allen and A. Bell, J. Am. Chem. Soc., 1942, 64, 1253. CrossRef
10. N. Vets, M. Smet, and W. Dehaen, Synlett, 2005, 217. CrossRef
11. A preliminary result has been reported, see: T. Takahashi, K. Kanno, and S. Li, WO 2011-JP55089, JP 2010-47726; Z. Jia, S. Li, K. Nakajima, K. Kanno, and T. Takahashi, J. Org. Chem., 2011, 76, 293. CrossRef
12. H. M. Qu, W. B. Cui, J. L. Li, J. J. Shao, and C. Y. Chi, Org. Lett., 2011, 13, 924. CrossRef
13. E. Clar and J. W. Wright, Nature, 1949, 163, 921. CrossRef
14. D. R. Coulson, L. C. Satek, and S. O. Grim, Inorg. Synth., 1972, 13, 121. CrossRef
15. K. Ono, H. Totani, T. Hiei, A. Yoshino, K. Saito, K. Eguchi, M. Tomura, J. Nishida, and Y. Yamashida, Tetrahedron, 2007, 63, 9699. CrossRef
16. J. Wang, K. Liu, Y. Liu, C. Song, Z. Shi, J. Peng, H. Zhang, and X. Cao, Org. Lett., 2009, 11, 2563. CrossRef
17. J. E. Anthony, D. L. Eaton, and S. R. Parkin, Org. Lett., 2002, 4, 15. CrossRef
18. J. E. Anthony, J. S. Brooks, D. L. Eaton, and S. R. Parkin, J. Am. Chem. Soc., 2001, 123, 9482. CrossRef