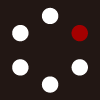
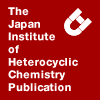
HETEROCYCLES
An International Journal for Reviews and Communications in Heterocyclic ChemistryWeb Edition ISSN: 1881-0942
Published online by The Japan Institute of Heterocyclic Chemistry
e-Journal
Full Text HTML
Received, 10th November, 2013, Accepted, 24th April, 2014, Published online, 12th May, 2014.
DOI: 10.3987/COM-13-12882
■ Two New Clerodane Diterpenoids from Croton crassifolius
Wei-Huan Huang, Guo-Qiang Li, Jia-Gui Li, Xia Wu, Wei Ge, Hau Yin Chung, Wen-Cai Ye, Yao-Lan Li,* and Guo-Cai Wang*
Institute of Traditional Chinese Medicine and Natural Products, College of Pharmacy, Jinan University, 601 West Huangpu Avenue, Guangzhou, 510632, China
Abstract
The root of Croton crassifolius was evaluated for its anti-angiogenic properties and anti-angiogenic components. The ethanol extract of the herb and its successive four subfractions were investigated in zebrafish model by quantitative endogenous alkaline phosphatase assay. Both petroleum ether (PE) and ethyl acetate fractions (EtOAc) showed potent anti-angiogenic activities. Two new clerodane diterpenoids, crassifolin I (1) and crassifolin H (2) were isolated from EtOAc fraction. Their structures were elucidated by extensive spectroscopic methods (IR, UV, HRESIMS, 1D and 2D NMR), and compound 1 was confirmed by single-crystal X-ray diffraction analysis. The further activities study showed that crassifolin H possessed anti-angiogenic activity by reducing vessel formation to 59.3% of the control value at concentrations of 15 μg/mL.INTRODUCTION
The roots of C. crassifolius Geisel. (Euphorbiaceae), a well-known traditional Chinese medicine (known as jiguxiang), are distributed mainly in south and southwest China, Laos, Thailand, and Vietnam, and are mainly used for the treatment of stomachache, rheumatism, sore throat, and cancer.1-3 Angiogenic diseases such as diabetic retinopathy, tumor growth and metastasis, rheumatoid arthritis, and inflammatory diseases would be caused by persistent unregulated angiogenesis, and the inhibition of angiogenesis is considered as a promising anticancer therapeutic strategy.4,5 Nevertheless, the anti-angiogenic effects of C. crassifolius have not been investigated. Because of its antirheumatism, and antitumor effects, the potential anti-angiogenic properties of C. crassifolius were investigated using wild type zebrafish in vivo model by quantitative endogenous alkaline phosphatase (EAP) assay in our study. Both petroleum ether (PE) and ethyl acetate (EtOAc) fractions from ethanol (EtOH) extract of the herb showed potent anti-angiogenic activities. Two new clerodane diterpenoids, crassifolin I (1) and crassifolin H (2) (Figure 1) were isolated from EtOAc fraction. Structures of the compounds were elucidated by spectroscopic methods and by comparison with closely related compounds. In the case of compound 1, the structure was confirmed by single-crystal X-ray diffraction analysis. The isolated compounds were further evaluated for their anti-angiogenic activities, and crassifolin I showed little anti-angiogenic effect, while crassifolin H possessed potent anti-angiogenic activity. Herein, this article reports the isolation and structural elucidation of the new clerodane diterpenes, as well as the anti-angiogenic activities investigation of C. crassifolius.
RESULTS AND DISCUSSION
Compound 1 was obtained as a colorless crystal and its molecular formula was analyzed as C20H22O6 by an [M+H]+ ion peak at m/z 359.1490 (Calcd for C20H23O6, 359.1489) in the HR-ESI-MS spectrum. The IR spectrum indicated the presence of hydroxyl (3443 cm-1) and carbonyl group (1752 cm-1). The UV spectrum showed absorption maxima at 211 nm. The signals at δH 6.48, 7.49, and 7.50 (1H each) in the 1H NMR spectrum were characteristics of a β-substituted furan ring. The signal of one methyl at δH 1.08 (3H, d, J = 6.9 Hz) was also displayed by the 1H NMR spectrum. The 13C NMR spectrum showed the presence of twenty carbon signals, including one methyl carbon at δC 15.6 and four furan ring carbons at δC 109.3 (C-14), 128.2 (C-13), 140.5 (C-16), 145.8 (C-15). The 1H and 13C NMR spectra of 1 showed a number of similarities to those of penduliflaworosin,6 except that the signals of C-4 (δC 47.5), C-19 (δC 22.8), C-6 (δC 26.6) and a methoxyl carbon (δC 51.9) in penduliflaworosin were replaced by the signals of a quaternary carbon (δC 50.0), a methylene carbon bearing oxygen (δC 67.7) and a methine carbon bearing oxygen (δC 77.2) in 1. It was reasonable to suppose that a lactone ring was formed between C-4 and C-6 on the basis of the HMBC correlation from H-6 (δH 5.23) to C-18 (δC 181.4) (Figure 2). The HMBC correlations from H-19 to C-3, C-4, C-5, C-18, and from H-3 to C-19 indicated that an additional hydroxyl group was attached to C-19. The configuration of 1 was elucidated based on the NOE interactions (H-6/H-17; H-6/H-19; H-8/H-11) (Figure 2). The signal assignments were completed by analysis of HSQC, HMBC, and 1H-1H COSY correlations. Furthermore, the structure of 1 was confirmed by a single-crystal X-ray diffraction analysis (Figure 2). Thus, the structure of 1 was elucidated as shown and was named as crassifolin I.
The molecular formula of compound 2 was determined as C19H20O5 by HR-ESI-MS ion peak at m/z 329.1383 [M+H]+ (Calcd for C19H21O5, 329.1384) in the spectrum. The IR spectrum showed the absorptions of ester carbonyl group at 1747, 1212 cm-1, and furan ring at 3140, 1445, 877 cm-1. The 1H NMR spectrum revealed the presence of one methyl [δH 1.11 (3H, d, J = 6.8 Hz)], and one β-substituted furan ring [δH 6.39, 7.43, and 7.46 (1H each, H-14, H-15, and H-16)]. The 13C NMR spectrum displayed the presence of nineteen carbon signals, including one methyl carbon at δC 16.4 and four furan ring carbons at δC 108.2 (C-14), 124.8 (C-13), 139.9 (C-16), 144.5 (C-15). The 1H and 13C NMR spectra of 2 were very similar to those of compound 1, except that the signals assigned to C-4 (δC 50.0) and C-19 (δC 67.7) of compound 1 were missing, and an additional methine at δC 39.7 was found in compound 2. This led to the hypothesis of the absence of the hydroxymethyl carbon C-19, and this was confirmed by HR-ESI-MS (C19H20O5 vs C20H22O6) and by the HMBC correlations between H-4 and C-5, C-10, and between H-2, H-3 and C-4. The configuration of 2 was elucidated as crassifolin D7 based on the NOE interactions of H-4/H-6, H-8/H-11 and H-12/H-17. Thus, compound 2 was deduced and named as crassifolin H.
The anti-angiogenic effects of C. crassifolius were examined in zebrafish in vivo model. The ethanol extract of the herb and its successive four subfractions (PE, EtOAc, n-butanol (BuOH), aqueous (Aq) fraction) were investigated by quantitative EAP assay. As showed in Figure 3A, the EtOH extracted, PE, and EtOAc fractions showed dose-dependently inhibited vessel formation. Compare to control, after the embryos treated with the EtOH, PE, and EtOAc fractions at concentration of 15 μg/mL, and the vessel formation were significantly (p < 0.05) reduced to 74.8, 69.4, and 62.8% of the control value, respectively. However, BuOH and Aq fractions did not show significant activities at all testing concentrations. The findings indicated the PE and EtOAc fractions were the effective fractions of C. crassifolius. Therefore, the compounds (crassifolin I and crassifolin H) isolated from EtOAc fraction were submitted to evaluate their anti-angeiogenic effects. In figure 3B, compare to control, crassifolin I showed little anti-angeiogenic effects, while crassifolin H dose-dependently inhibited vessel formation. After the embryos treated with crassifolin H at final concentrations of 3.25, 7.5, and 15 μg/mL, and the vessel formation were significantly (p < 0.05) reduced to 91.4, 80.4, and 59.3% of the control value, respectively.
Previous phytochemical research of C. crassifolius resulted in the isolation of a series of clerodane diterpenoids.7-10 As part of our studies on C. crassifolius, this paper reports the isolation and structure identification of two new clerodane diterpenoids, crassifolin I and crassifolin H. And our bioactivity data provide the first evidence that C. crassifolius possessed anti-angeiogenic properties and diterpenoids was one of the active components because crassifolin H possessed potent anti-angeiogenic effect. However, crassifolin I with similar chemical structure to crassifolin H (Figure 1) showed little anti-angeiogenic effect. The results indicated that different substitution of functional groups can affect the anti-angeiogenic activity. Clerodane diterpenoids are the main bioactive constituents in C. crassifolius and a series of clerodane diterpenoids have been isolated from the herb,7-10 however, their anti-angeiogenic activities have rare been reported. Thus, further investigation will be necessary to study the anti-angeiogenic effect and mechanism of the other clerodane diterpenoids in this herb, and to deduce their structure-activity relationship.
EXPERIMENTAL
General. Melting points were measured on an X-5 micro melting point apparatus (Tech, Beijing, P. R. China). Optical rotations were determined by a Jasco P-1020 digital polarimeter. UV spectra were recorded on a Jasco V-550 UV/VIS instrument. A Jasco FT/IR-480 plus spectrometer was used for scanning the IR spectra with KBr pellets. 1D and 2D NMR spectra were recorded on a Bruker AV-400 spectrometer with TMS as internal standard, and chemical shifts (δ) were expressed in ppm with reference to the solvent signals. HR-ESI-MS were determined on an Agilent 6210 ESI/TOF mass spectrometer. Column chromatography (CC) was performed with silica gel (200-300 mesh, Qingdao Marine Chemical Plant, Qingdao, P. R. China) or Sephadex LH-20 (Pharmacia Biotech, Uppsala, Sweden). TLC was performed on precoated silica gel GF254 plates (Yantai Chemical Industry Research Institute, Yantai, China). Preparative HPLC was performed on an Agilent system equipped with a preparative Cosmosil C18 (20 × 250 mm) column. EAP staining was assayed with phosphatase substrate kit (Pierce, Rockford, USA).
Plant Material. The roots of C. crassifolius were collected in Conghua City, Guangdong province of China, in May of 2011, and were authenticated by Professor Guang-Xiong Zhou of Jinan University. A voucher specimen (No. 2011051712) was deposited in the Institute of Traditional Chinese Medicine and Natural Products, Jinan University, Guangzhou, P. R. China.
Extraction and Isolation. The dried and powdered roots of C. crassifolius (5.0 kg) were percolated with 95% EtOH at room temperature. Removal of the EtOH from the extract under reduced pressure yielded a residue (465 g) which was then suspended in H2O and extracted successively PE, EtOAc and BuOH, leaving a residual aqueous (Aq) fraction. Each subfraction was evaporated under reduced pressure to yield the extracts of PE, EtOAc, BuOH, and Aq fractions, respectively. The EtOAc extract (76 g) was chromatographed on a silica gel column with gradient mixtures of petroleum ether-acetone (100:1 → 100:50). Ten fractions were collected and analyzed by TLC. Fraction 3 was re-chromatographed on silica gel (petroleum ether-EtOAc, 100:7 → 100:15) to give subfractions 8-1 to 8-5. Subfraction 8-5 was separated over silica gel (petroleum ether-EtOAc, 100:10 → 100:25) and further purified by RP-HPLC (60% MeOH/H2O) to afford compound 2 (15 mg). Fraction 10 was subjected to a silica gel CC (petroleum ether-acetone, 100:1 → 10:8) to give subfractions 10-1 to 10-4. Subfraction 10-2 was crystallized from acetone-MeOH to afford compound 1 (5 mg).
Crassifolin I (1): colorless crystals (MeOH); mp 258-265 °C; [α]25D +117.0 (c 1.1, MeOH); UV (MeOH) λmax: 211 (0.81) nm; IR (KBr) νmax: 3443, 1752, 1336, 1036, 741 cm-1 ; 1H and 13C NMR data, see Table 1; HR-ESI-MS m/z 359.1490 [M + H]+ (calcd for C20H23O6, 359.1489).
Crassifolin H (2): white amorphous powder; [α]25D + 66.7 (c 1.2, MeOH); UV (MeOH) λmax: 215 (1.07) nm; IR (KBr) νmax: 3140, 1747, 1672, 1445, 1212, 1036, 877 cm-1 ; 1H and 13C NMR data, see Table 1; HR-ESI-MS m/z 329.1383 [M + H]+ (calcd for C19H21O5, 329.1384).
X-Ray crystallography of 1. A colorless blocks crystal suitable for X-ray analysis was recrystallized from MeOH. The X-ray data of compound 1 were collected at 293(2) K with Cu Kα radiation ( λ = 1.5418 Å) on an Agilent Gemini S Ultra CCD diffractometer. Crystal data: C20H22O6 (fw = 358.14), crystal dimensions 0.28 × 0.24 × 0.20 mm, monoclinic, space group P21, a = 15.1870(6) Å , b = 6.7319(2) Å , c = 17.4007(7) Å, β = 101.540(4)°, V = 1743.03(12) Å3, Z = 4, DC = 1.366 mg/mm3, F(000) = 760. A total of 7374 reflections were collected in the range 2.97 ≤ θ ≤ 60.79, of which 4110 unique reflections with I > 2σ(I) were collected for the analysis. The structure was refined by full-matrix least squares on F2 using SHELXL-97 package software.11 The final reliability factors are: R = 0.0328, RW = 0.0870, and the goodness of fit on F2 was equal to 1.098. Crystallographic data for the structure reported in this paper have been deposited at the Cambridge Crystallographic Data Centre under the reference number CCDC 949710.
Quantitative EAP assay on zebrafish embryo. The wild type zebrafish of AB strain was used. For experiment, the zebrafish embryos were used and the embryos were cultured in embryo water (0.2 g/L Instant Ocean Salt) at 28.5 °C. During zebrafish development, the stage between 24 h post-fertilization (hpf) and 72 hpf has the highest angiogenic activity and EAP staining was assayed with phosphatase substrate kit according to the method of He et al.12 Zebrafish embryos (24 hpf) were manually dechorionated with forceps and arrayed in a 96-well plate (one embryo per well) and incubated with 100 μL of embryo water per well containing various concentrations of samples, while 0.2% dimethylsulfoxide (DMSO) was set as control. After further incubation at 28.5 °C for 48 h, embryos were washed and stained according to the instruments in phosphatase substrate kit. After staining, 50 μL of the 2M NaOH was added to stop the reaction. The optical densities were determined at the wavelength of 405 nm. Vessel growth was presented as percentage formation of vessel in optical density comparing to control [% vessel formation = (OD sample day 3 − OD control day 1) / (OD control day 3 − OD control day 1) × 100%].
Statistical analysis. The quantitative EAP assay on zebrafish embryo among the samples was run in triplicate. Data were expressed as means ± standard deviation (n = 10). Data were evaluated for significance with the one-way Analysis of Variance (ANOVA) procedure followed by Dunnett’s test. P values less than 0.05 were considered significant.
ACKNOWLEDGEMENTS
This work was supported financially by the Natural Science Foundations of China (81202429 and 81273390), the Fundamental Research Funds for the Central Universities (21612417) and the China Postdoctoral Science Foundation (2012M521656).
References
1. H. X. Qiu, Flora Republicae Popularis Sinicae, Science Press, Beijing, 1996, p. 130.
2. Chinese Materia Medica Editorial Committee, Chinese Materia Medica, Shanghai Scientific and Technical Publisher, Shanghai, 1999, p. 767.
3. L. Boonyarathanakornkit, C. T. Che, H. H. S. Fong, and N. R. Farnsworth, Planta Med., 1988, 54, 61. CrossRef
4. J. Folkman, N. Engl. J. Med., 1971, 285, 1182. CrossRef
5. J. Folkman, Nat. Med., 1995, 1, 27. CrossRef
6. H. M. Zakaria, F. Kenne, C. C. Musa, C. K. Modest, J. M. Joseph, M. M. Mainen, L. Filip, G. Kees, F. Jan, P. Rene, V. Arnold, A. Sandra, and P. Luc, Planta Med., 2009, 75, 262. CrossRef
7. G. C. Wang, J. G. Li, G. Q. Li, J. J. Xu, X. Wu, W. C. Ye, and Y. L. Li, J. Nat. Prod., 2012, 75, 2188. CrossRef
8. S. H. Chen, F. Z. Ren, L. H. Li, Y. Q. Gao, and N. Wang, Chin. Pharm. J., 2010, 45, 1907.
9. X. H. Yang, S. W. Chen, and S. M. Deng, Shizhen Guoyi Guoyao., 2009, 20, 515.
10. X. H. Yang, S. W. Chen, Q. Lin, and S. M. Deng, Guihaia., 2009, 29, 272.
11. G. M. Sheldrick, Acta Cryst., 2008, A64, 112. CrossRef
12. Z. H. He, M. F. He, S. C. Ma, and P. P. H. But, J. Ethnopharmacol., 2009, 121, 313. CrossRef