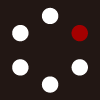
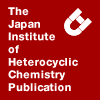
HETEROCYCLES
An International Journal for Reviews and Communications in Heterocyclic ChemistryWeb Edition ISSN: 1881-0942
Published online by The Japan Institute of Heterocyclic Chemistry
e-Journal
Full Text HTML
Received, 27th February, 2014, Accepted, 25th March, 2014, Published online, 4th April, 2014.
DOI: 10.3987/COM-14-S(K)14
■ Phenol and Aniline Oxidative Coupling with Alkenes by Using Hypervalent Iodine Dimer for the Rapid Access to Dihydrobenzofurans and Indolines
Toshifumi Dohi, Yosuke Toyoda, Tomofumi Nakae, Daichi Koseki, Hiroko Kubo, Tohru Kamitanaka, and Yasuyuki Kita*
College of Pharmaceutical Sciences, Ritsumeikan University, 1-1-1 Nojihigashi, Kusatsu, Shiga, 525-8577, Japan
Abstract
A highly reactive hypervalent iodine dimer, [Ph(CF3COO)I]-O-[I(OCOCF3)Ph], is utilized as successful promoter in the oxidative phenolic coupling with styrenes leading to 2-aryldihydrobenzofurans. The extensions of the substrates in this study have led to the development of a new expeditious construction of the pyrroloindoline structure from aniline and enamide derivatives.Phenolic oxidation is one of the pivotal steps frequently involved during the biosynthesis of naturally- occurring products possessing a variety of interesting biological activities.1 Consequently, there already exist continuing efforts in chemical processes for reproducing such transformations, and hypervalent iodine reagents, specifically, PhI(OAc)2 [phenyliodine diacetate, PIDA] and PhI(OCOCF3)2 [phenyl iodine bis(trifluoroacetate), PIFA], are found to be versatile synthetic tools for producing the valuable quinone, quinol, and spirodienone-type compounds through the biomimetic phenolic oxidations under mild conditions.2 In addition, many favorable characteristics, i.e., low toxicity, safety, ready availability, easy handling, etc., have made these iodine reagents a useful and essential class as the oxidation tool in modern organic synthesis developing the green chemistry that can replace toxic heavy-metal oxidizers, such as lead, mercury, and thallium-based reagents.3 Over the past two decades, we have thoroughly investigated the development of a series of hypervalent iodine-induced oxidative transformations accompanying the dearomatizations of phenols and related compounds by exploiting a wide array of new reactivities and synthetic utility, and the products obtained by these protocols were utilized as key intermediates for accomplishing the total syntheses of several complex natural products and related functionalized molecules having interesting biological properties.4
In spite of the increased chemistry in the phenolic oxidation field of hypervalent iodine reagents, the transformations accompanying some carbon-carbon bond formations were, on the other hand, only slightly reported, especially involving the intermolecular reactions.5-9 One of the early examples concerning this topic is the oxidative phenolic coupling with electron-rich styrenes to produce dihydro- benzofuran products by the formal cycloaddition sequence.9,10 As the primary research, Swenton and co-workers reported the reactions of a few combinations of p-methoxyphenols 1 with p-methoxystyrene derivatives using PhI(OCOCF3)2 in acetonitrile (Scheme 1).9 A generally accepted reaction mechanism includes the initial attack of the phenolic oxygen atom on the iodine center to form the phenoxyiodine(III) intermediate. For the electron-rich phenols 1 having the cation-stabilizing methoxy group, this intermediate would produce discrete quinonium ions in a polar solvent, such as acetonitrile, by the smooth dissociation of iodobenzene and trifluoroacetic acid. The charged cationic species can now react with the electron-rich styrenes 2 to afford the keto-type pre-cyclized intermediate, which is simultaneously converted to the final dihydrobenzofuran products 3 by aromatization as the driving force. The stepwise constructions of the carbon-carbon and carbon-oxygen bonds between the phenols 1 and styrenes 2 would typically deliver the thermodynamically-favored cyclic isomers. This method could indeed provide the corresponding dihydrobenzofuran products 3 in only one-step from these readily available substrates, but it usually suffered from low yield formations of the products, thus still has significant room for improvement.
During our continuous investigations of hypervalent iodine chemistry, we recently met the remarkable high reactivities of hypervalent iodine dimers linked to each other via the oxygen bridge.11,12 Interestingly, it was obvious that an alternative use of the PIFA dimer, i.e., [Ph(CF3COO)I]O[I(OCOCF3)Ph], instead of PhI(OCOCF3)2 itself, can generally further enhance the productivities of a variety of typical phenolic oxidation processes using a hypervalent iodine reagent.12 A plausible explanation of the high reactivity is that the more polarized I(III)-OCOCF3 bonds compared to those of PhI(OCOCF3)213 would significantly develop the positive characters of the iodine atoms in order to smoothly interact with the phenolic oxygen and substrates. This encouraged us to examine in this study the PIFA dimer as a superior candidate in the reported oxidative coupling of phenols as well as anilines 1 toward styrenes 2 leading to the dihydro- benzofuran and indoline products 3.9,10 As our continuous focus on these types of µ-oxo bridged hypervalent iodine reagents, herein, we now report initial experimental results using the PIFA dimer in this transformations regarding the representative substrates, which have partial improvements in the yields of the obtained benzoheterocycles that are higher than those by the conventional PhI(OCOCF3)2 used to synthesize the 2-aryldihydrobenzofurans.14 With a highly reactive reagent, the rapid access to the physostigmine-type cyclic pyrroloindoline structure is also demonstrated by employing a combination of the suitably protected anilide and cyclic enamide as an extension of the oxidative coupling strategy.
The PIFA dimer 1 is a colorless solid (mp 108-111 oC) not sensitive to air and moisture, which is thus stable under protection from light and allows long-term storage in a refrigerator. Its preparation requires only one-step from a commercially available material, and in this study, the high purity PIFA dimer can be obtained in good yields from iodosobenzene (PhIO) by precipitation after the reaction with a stoichiometric amount of trifluoroacetic acid in refluxing chloroform or alternatively, from the corresponding monomer, that is, PIFA, by treatment with an equimolar base, such as potassium tert- butoxide.13 This compound is readily soluble in various media, even in hot water, and the reactions can be performed in many organic solvents.
For comparison of the reactivities, our evaluation of the PIFA dimer during the phenolic oxidative coupling with alkenes was started in the literature with the combination of substrates (Scheme 2). Thus, it is known that the treatment of 4-methoxyphenol 1a and 4-vinyl anisole 2a with PhI(OCOCF3)2 produced the 2-aryldihydrobenzofuran 3aa (corsifuran A) in 57% yield in acetonitrile after a detailed optimization of the conditions.9 Similarly, we examined the same conversion of the substrates using the PIFA dimer. An equimolar mixture of phenol 1a and styrene 2a in acetonitrile was thus treated with the PIFA dimer under the same conditions for the same reaction time (see Experimental Section). We employed the dimer oxidant in a 0.5 equivalent relative to the substrates considering the presence of the two active iodine sites in the reagent molecule. In this case, our performance of the reaction, to our delight, the apparent improvement of the isolated product was 76% yield after the same workup, which corresponds to about a 20% increase in the product yield in comparison to the reference work using the optimized method with the conventional PhI(OCOCF3)2 reagent. In other higher or lower concentrations, the product 3aa was also obtained in similar good yields at both 0 °C and room temperature.
With this encouraging result, we next attempted to apply the oxidative coupling cyclizations using the PIFA dimer with several other phenol (Eq. 1) and alkene (Eq. 2) substrates. Using the same procedures, the reactions of the ortho-substituted phenols 1b and 1c having a bulky substituent or a weak electron-withdrawing functionality toward the styrene 2a could afford the dihydrobenzofurans 3ba and 3ca in moderate yields. The decreased yield would suggest that the reactions are relatively sensitive to both the steric and electronic effects of the ortho groups in the phenolic substrates. In addition, the β- substituent at the neighboring reaction site also seemed to influence the reaction progress. Thus, the 3,5- dimethylphenol 1d produced the corresponding 4,6-disubstituted benzofuran 3da also in a moderate yield. The phenol structure is more suitable for this reaction based on the result using the naphthol substrate 1e, probably due to the undesired facile SET-oxidation sensitivity of the naphthalene ring.15 The reactions were usually fast enough to be completed within half an hour.
Regarding the alkene coupling partners 2, p-alkylstyrene 2b similarly reacted with the reference phenol 1a in an expected manner to form the product 3ab. On the other hand, the conformationally restricted 2- mesitylbenzofuran 3ac was obtained in a lower yield (53%) by the reaction of vinyl mesitylene 2c. As the disubstituted alkenes, anethole 2d and α-methylstyrene 2e were employed to give the corresponding cyclized products 3ad and 3ae in 76% and 63% yields, respectively, by the oxidative couplings. From the trans and cis-mixture 2d, the product 3ad was obtained as a single trans-isomer.
Despite the low reaction yield, the standard styrene 2f could be coupled with the phenol 1a (Scheme 3). For such a less reactive alkene, the use of a more polar solvent instead of acetonitrile effectively improved the yield to some extent. Specifically, some fluoroalcohols can support the generation of the cationic reaction intermediates by solvation throughout the reactions.16 To this end, the product 3af was formed in 50% yield in 2,2,2-trifluoroethanol from the styrene 2f and the phenol 1a.
Besides, this reaction has an alternative synthetic route toward the construction of the indoline structures. Accordingly, the method was applied to the suitably modified aniline derivatives, and it was clarified that the N-tosylanilide 1f could also more smoothly combine with anethole 2d into the indoline product 3fd (Scheme 4). Note that the yield was pleasantly higher when using the PIFA dimer (versus 83% by PhI(OCOCF3)2 in our previous report under the same conditions10). Thus, the oxidative coupling in the presence of excess alkene 2d (3 equiv) in dichloromethane with 2.5 x 1/2 equiv. of the PIFA dimer afforded the indoline 3fd in the excellent yield of 93%.
It is of particular importance that some naturally-occurring biologically active compounds belonging to the pyrroloindoline families consist of the lead molecules in modern drug developments.17 The above- demonstrated successful result in producing the indoline structure strongly motivated us to examine the cyclic enamide as an extension of the alkene component in combination with the PIFA dimer for a new one-step access to the pyrroloindoline structure. To validate, we confirmed that the typical procedure in Scheme 4 allowed the oxidative coupling of the anilide 1f with N-tosyl cyclic enamide 2g (Scheme 5), resulting in detection of the target bicyclic indoline 4. The starting anilide 1f disappeared after the reaction. Thus, the low yield of the product 4 seems to be caused by the too low nucleophilicity of enamide 2g as a coupling partner. This first outcome of the direct construction of the pyrroloindoline structure from the aniline substrate might expect future progress of the method in this study as an expeditious approach toward a series of physostigmine-type compounds.18
The PIFA dimer employed in this study was revealed as a new good promoter for the oxidative couplings of phenols with styrenes as well as anilides. The high reactivity is suitable for promoting the coupling cyclization to 2-aryldihydrobenzofurans and indolines by directly furnishing the carbon-carbon and carbon-oxygen or carbon-nitrogen bonds. Its utilization has led to some extensions of the reaction substrates, which is featured in a partial success of the direct and one-step construction of the physostigmine-type cyclic pyrroloindoline structure from the corresponding anilide and cyclic enamide.
EXPERIMENTAL
Melting point (mp) is uncorrected. All 1H- and 13C-NMR spectra of the obtained products were measured in CDCl3 by NMR spectrometers operating at 400 MHz (100 MHz for 13C NMR) at 25 °C. Chemical shifts of 1H-NMR were recorded in parts per million (ppm, δ) relative to tetramethylsilane (δ = 0.00 ppm) as an internal standard. Data are reported as follows: chemical shift in ppm (δ), multiplicity (s = singlet, d = doublet, t = triplet, m = multiplet), coupling constant (J) in Hz, and integration. Chemical shifts of 13C-NMR were reported in ppm with the solvent as reference peak (CDCl3: δ = 77.0 ppm). High resolution mass measurements were performed by the Elemental Analysis Section of Osaka University. Flash column chromatography was performed with Merck Silica Gel 60 (230-400 mesh) eluting with hexane and ethyl acetate for isolation of the products. Analytical thin-layer chromatography (TLC) was carried out on Merk Silica Gel F254 plates (0.25 mm). The spots and bands were detected by UV light of irradiation (254, 365 nm) and/or by staining with 5% phosphomolybdic acid followed by heating. Unless otherwise noted, all experiments were carried out at room temperature in open flask without care of moisture.
Materials
The protected aniline 1f was prepared by the standard protection method of 4-methoxyaniline with p- toluenesulfonyl chloride under basic conditions. The cyclic enamide 2g was obtained according to the literature.19 All other chemicals and solvents for the experiments and chromatography are commercially available and used as received in this study without further purification.
Gram-Scale preparation of µ-oxodiphenylbis(trifluoroacetato-O)diiodine (PIFA dimer)
The preparation was performed by a modified synthetic procedure reported in the literature.13 Thus, to a suspension of the iodosobenzene polymer (PhIO, 2.20 g, 10 mmol) in CHCl3 (75 mL) was added an equimolar amount of trifluoroacetic acid (1.14 g, 10 mmol) and the resulting mixture was refluxed for about 2 h. The reaction mixture was filtered and the solvent of the filtrate was evaporated. The residue in a small amount of CHCl3 was triturated by adding hexane to precipitate the PIFA dimer (4.88 g, 75%) as a colorless solid (mp 108-111 °C [lit.,13 mp 110-112 °C]).
Another preparation method was the treatment of PhI(OCOCF3)2 with a half equimolar amount of potassium tert-butoxide in chloroform at room temperature for 1 h followed by a similar workup. The PIFA dimer was obtained in 97% yield by this alternative method on the same scale.
Typical procedure for the oxidative coupling of phenols 1 with styrenes 2 using the PIFA dimer leading to dihydrobenzofurans 3 (Schemes 2 and 3, Eqs 1 and 2)
To an ice-cooled solution of equimolar phenol 1a (24.8 mg, 0.20 mmol) and styrene 2a (27.6 mg, 0.20 mmol) in MeCN (0.5 mL), the PIFA dimer (65.0 mg, 0.10 mmol) was added in one portion at room temperature. The reaction mixture was stirred for 30 min and then it was quenched with solid sodium hydrogen carbonate. After filtration, solvent was removed in vacuo and the residue was purified by column chromatography on silica-gel to give 2-aryldihydrobenzofuran 3aa (40.1 mg, 0.152 mmol) in 76% yield. We also confirmed the reaction in semi-gram scales.
2,3-Dihydro-5-methoxy-2-(4-methoxyphenyl)benzofuran (3aa, corsifuran A)9a,b
Colorless solid; 1H-NMR (400 MHz, CDCl3): 3.10 (dd, J = 16.5, 8.8 Hz, 1H), 3.45 (dd, J = 16.5, 8.8 Hz, 1H), 3.70 (s, 3H), 3.75 (s, 3H), 5.62 (t, J = 8.8 Hz, 1H), 6.56-6.73 (m, 4H), 6.78-6.86 (m, 2H), 7.22-7.33 (m, 2H) ppm; 13C-NMR (100 MHz, CDCl3): 38.7, 55.3, 56.0, 84.2, 109.2, 111.1, 113.0, 113.9, 127.3, 127.7, 133.9, 153.7, 154.2, 159.4 ppm.
The physical and spectral data of the products 3aa, 3ea, 3ad, 3ae, and 3af matched those previously reported.
7-tert-Butyl-2,3-dihydro-5-methoxy-2-(4-methoxyphenyl)benzofuran (3ba)
Slightly orange-coloured oil; 1H-NMR (400 MHz, CDCl3): 1.37 (s, 3H), 3.09 (dd, J = 15.6, 8.8 Hz, 1H), 3.52 (dd, J = 15.6, 9.3 Hz, 1H), 3.76 (s, 3H), 3.80 (s, 3H), 5.66-5.71 (m, 1H), 6.62 (d, J = 2.4 Hz, 1H), 6.70 (d, J = 2.9 Hz, 1H), 6.89 (d, J = 8.8 Hz, 2H), 7.32 (d, J = 8.8 Hz, 2H) ppm; 13C-NMR (100 MHz, CDCl3): 29.2, 34.2, 39.0, 55.2, 55.9, 83.3, 107.2, 111.5, 113.8, 126.9, 127.4, 133.4, 134.9, 151.6, 153.8, 159.1 ppm; HRMS (EI): calcd for C20H24O3 (M)+: 312.1725, found 312.1720.
7-Chloro-2,3-dihydro-5-methoxy-2-(4-methoxyphenyl)benzofuran (3ca)
Colorless oil; 1H-NMR (400 MHz, CDCl3): 3.23 (dd, J = 15.6, 8.3 Hz, 1H), 3.59 (dd, J = 15.6, 9.3 Hz, 1H), 3.73 (s, 3H), 3.79 (s, 3H), 5.74 (t, J = 8.8 Hz, 1H), 6.66-6.70 (m, 2H), 6.88 (d, 2H, J = 8.8 Hz), 7.31 (d, J = 8.8 Hz, 2H) ppm; 13C-NMR (100 MHz, CDCl3): 39.3, 55.3, 56.1, 84.7, 110.1, 113.0, 114.0, 114.3, 127.4, 129.0, 133.1, 149.8, 154.4, 159.5 ppm; HRMS (EI): calcd for C16H15ClO3 (M)+: 290.0710, found 290.0704.
2,3-Dihydro-5-methoxy-4,6-dimethyl-2-(4-methoxyphenyl)benzofuran (3da)
Slightly brown-coloured oil; 1H-NMR (400 MHz, CDCl3): 2.15 (s, 3H), 2.24 (s, 3H), 3.05 (dd, J = 15.6, 8.3 Hz, 1H), 3.45 (dd, J = 15.2, 9.3 Hz, 1H), 3.66 (s, 3H), 3.79 (s, 3H), 5.66 (m, 1H), 6.48 (s, 1H), 6.88 (d, J = 8.8 Hz, 2H), 7.31 (d, J = 8.8 Hz, 2H) ppm; 13C-NMR (100 MHz, CDCl3): 13.2, 16.5, 37.8, 55.3, 60.2, 84.1, 108.3, 113.9, 124.3, 127.2, 127.3, 130.2, 134.2, 150.7, 155.0, 159.4 ppm; HRMS (EI): calcd for C18H20O3 (M)+: 284.1412, found 284.1407.
2,3-Dihydro-5-methoxy-2-(4-methoxyphenyl)naphtha[1,2-b]furan (3ea)9b
Colorless solids; 1H-NMR (400 MHz, CDCl3): 3.34 (dd, J = 15.6, 7.8 Hz, 1H), 3.74 (dd, J = 15.6, 7.8 Hz, 1H), 3.79 (s, 3H), 3.94 (s, 3H), 5.86 (t, J = 15.6 Hz, 1H), 6.72 (s, 1H), 6.87-6.90 (m, 2H), 7.36-7.48 (m, 4H), 7.92-7.94 (m, 1H), 8.17-8.20 (m, 1H) ppm; 13C-NMR (100 MHz, CDCl3): 40.0, 55.2, 56.0, 84.0,
101.5, 113.9, 118.1, 120.7, 121.2, 122.4, 124.9, 125.3, 126.0, 127.2, 134.5, 148.3, 150.0, 159.3 ppm.
2-(4-tert-Butylphenyl)-2,3-dihydro-5-methoxybenzofuran (3ab)
Colorless solids; mp 46-47 °C; 1H-NMR (400 MHz, CDCl3): 1.31 (s, 9H), 3.22 (dd, J = 15.6, 8.3 Hz, 1H), 3.56 (dd, J = 15.6, 9.3 Hz, 1H), 3.76 (s, 3H), 5.71 (t, J = 8.8 Hz, 1H), 6.68-6.78 (m, 3H), 7.33-7.40 (m, 4H) ppm; 13C-NMR (100 MHz, CDCl3): 32.1, 35.4, 39.4, 56.8, 85.0, 110.0, 112.0, 113.7, 126.4, 126.5, 128.5, 139.6, 151.9, 154.6, 155.0 ppm; HRMS (EI): calcd for C19H22O2 (M)+: 282.1620, found 282.1622.
2,3-Dihydro-2-mesityl-5-methoxybenzofuran (3ac)
Colorless oil; 1H-NMR (400 MHz, CDCl3): 2.26 (s, 3H), 2.30 (s, 6H), 3.20-3.27 (m, 1H), 3.38-3.45 (m, 1H), 3.77 (s, 3H), 6.08-6.13 (m, 1H), 6.68-6.73 (m, 2H), 6.79 (d, J = 1.0 Hz, 1H), 6.85 (s, 2H) ppm; 13C-NMR (100 MHz, CDCl3): 20.3, 20.8, 36.4, 56.0, 81.6, 109.4, 110.9, 113.1, 128.0, 130.0, 133.4, 136.6, 137.3, 153.7, 154.0 ppm; HRMS (EI): calcd for C18H20O2 (M)+: 268.1463, found 268.1458.
trans-2,3-Dihydro-5-methoxy-2-(4-methoxyphenyl)-3-methylbenzofuran (3ad)9a,b
Colorless oil; 1H-NMR (400 MHz, CDCl3): 0.88 (d, J = 8.0 Hz, 3H), 2.90-2.93 (m, 1H), 3.29 (s, 3H), 3.31 (s, 3H), 4.58 (d, J = 8.0 Hz, 1H), 6.18-6.30 (m, 3H), 6.40-6.45 (m, 2H), 6.86 (d, J = 8.0 Hz, 2H) ppm; 13C-NMR (100 MHz, CDCl3): 17.5, 45.6, 55.3, 56.0, 92.5, 109.3, 110.0, 112.8, 113.9, 127.6, 132.6, 133.0, 153.2, 154.4, 159.6 ppm.
2,3-Dihydro-5-methoxy-2-methyl-2-(4-methoxyphenyl)benzofuran (3ae)15e
Colorless oil; 1H-NMR (400 MHz, CDCl3): 1.74 (s, 3H), 2.32 (s, 3H), 3.45-3.27 (m, 2H), 3.74 (s, 3H), 6.74-6.63 (m, 2H), 6.78 (d, J = 8.8 Hz, 1H), 7.15 (d, J = 8.0 Hz, 2H), 7.35 (d, J = 8.0 Hz, 2H) ppm; 13C-NMR (100 MHz, CDCl3): 21.0, 29.2, 45.2, 56.0, 76.7, 77.0, 77.3, 89.2, 109.3, 111.3, 112.9, 124.5, 127.6, 129.0, 136.6, 143.9, 153.1, 154.1 ppm.
2,3-Dihydro-5-methoxy-2-phenylbenzofuran (3af)15f
Colorless oil; 1H-NMR (400 MHz, CDCl3): 3.16 (dd, J = 15.6, 8.3 Hz, 1H), 3.57 (dd, J = 15.6, 9.2 Hz, 1H), 3.73 (s, 3H), 5.70 (t, J = 8.8 Hz, 1H), 6.66-6.74 (m, 1H), 6.75-6.76 (m, 2H), 7.28-7.39 (m, 5H) ppm; 13C-NMR (100 MHz, CDCl3): 38.5, 55.6, 83.8, 108.8, 110.8, 112.6, 125.4, 127.1, 127.6, 128.2, 141.6, 153.4, 153.9 ppm.
Procedure for the oxidative coupling of aniline 1f with alkenes 2d and 2g using the PIFA dimer leading to indoline 3fd and it derivatives (Schemes 4 and 5)
To a stirred solution of anilide 1f (27.7 mg, 0.10 mmol) and anethole 2d (44.5 mg, 0.30 mmol) or cyclic enamide 2g (66.9 mg, 0.30 mmol) in CH2Cl2 (10 mL) was added dropwise a solution of the PIFA dimer (82 mg, 0.125 mmol) in CH2Cl2 (10 mL) over 2 h by the syringe pump at room temperature. The reaction mixture was then stirred for additional 1 h. The solvent was evaporated in vacuo and the residue was purified by column chromatography on silica-gel to give pure indoline 3fd (44.0 mg, 0.093 mmol) or pyrroloindoline 4 (11.0 mg, 0.022 mmol), respectively.
trans-2,3-Dihydro-5-methoxy-2-(4-methoxyphenyl)-3-methyl-1-[(4-methylphenyl)sulfonyl]-1H-indole (3fd)10
Colorless oil; 1H-NMR (400 MHz, CDCl3): 0.78 (d, J = 7.0 Hz, 3H), 2.36 (s, 3H), 3.00-3.04 (m, 1H), 3.77 (s, 3H), 3.78 (s, 3H), 4.60 (d, J = 3.7 Hz, 1H), 6.56 (d, J = 2.4 Hz, 1H), 6.78-6.84 (m, 3H), 7.18-7.25 (m, 4H), 7.59 (d, J = 8.2 Hz, 2H), 7.68 (d, J = 8.9 Hz, 1H) ppm; 13C-NMR (100 MHz, CDCl3): 21.5, 21.6, 46.1, 55.3, 55.6, 72.6, 110.1, 113.1, 113.9, 116.8, 127.0, 127.3, 129.4, 134.5, 134.6, 134.9, 137.6, 143.8, 157.1, 159.0 ppm.
1,2,3,3a,8,8a-Hexahydro-5-methoxy-1,8-di[(4-methylphenyl)sulfonyl]pyrrolo[2,3-b]indole (4)
Colorless solid; mp 72-75 °C; 1H-NMR (400 MHz, CDCl3): 1.87 (dd, J = 12.4, 4.9 Hz, 1H), 1.97-2.07 (m, 1H), 2.64-2.71 (m, 1H), 2.31 (s, 3H), 2.38 (s, 3H), 3.39 (m, 1H), 3.39 (t, J = 6.8 Hz, 1H), 3.70 (s, 3H), 3.70-3.75 (m, 1H), 6.19 (d, J = 6.6 Hz, 1H), 6.48 (d, J = 2.4 Hz, 1H), 6.67 (dd, J = 8.8, 2.7 Hz, 1H), 7.10 (d, J = 8.0 Hz, 2H), 7.22-7.27 (m, 3H), 7.44 (d, J = 8.3 Hz, 2H), 7.86 (d, J = 8.3 Hz, 2H) ppm; 13C-NMR (100 MHz, CDCl3): 21.5, 31.4, 46.5, 46.8, 55.5, 81.4, 109.5, 113.6, 119.1, 127.3, 127.5, 129.3, 129.5, 134.4, 134.7, 135.2, 137.4, 143.2, 144.0, 158.0 ppm; HRMS (EI): calcd for C25H26N2O5S2Na (M + Na)+: 521.1181, found 521.1175.
ACKNOWLEDGEMENTS
This work was supported by a Grant-in-Aid for Scientific Research (A) and Encouragement of Young Scientists (A) from the JSPS, a Grant-in-Aid for Scientific Research on Innovative Areas “Advanced Molecular Transformations by Organocatalysts” from MEXT, and the Ritsumeikan Global Innovation Research Organization (R-GIRO) Project. T.D. is grateful for financial support from the Asahi Glass Foundation and the Industrial Technology Research Grant Program of NEDO of Japan. N.Y. thanks the JSPS for Young Scientists for a research fellowship.
References
1. Selected recent reviews and books: (a) S. K. Jackson, K.-L. Wu, and T. R. R. Pettus, Biomimetic Organic Synthesis, ed. by E. Poupon and B. Nay, Wiley-VCH, Weinheim, 2011, Vol. 2, pp. 723-749; CrossRef (b) S. Yamamura, Chemistry of Phenols, ed. by Z. Rappoport, John Wiley & Sons, Chichester, 2003, Vol. 2, pp. 1153-1346; CrossRef (c) T. Pal and A. Pal, Curr. Sci., 1996, 71, 106.
2. For reviews and accounts, see: (a) R. M. Moriarty and O. Prakash, Org. React., 2001, 57, 327; (b) A. Pelter and R. S. Ward, Tetrahedron, 2001, 57, 273; CrossRef (c) M. A. Ciufolini, N. A. Braun, S. Canesi, M. Ousmer, J. Chang, and D. Chai, Synthesis, 2007, 3759; CrossRef (d) L. Pouységu, D. Deffieux, and S. Quideau, Tetrahedron, 2010, 66, 2235; CrossRef (e) H. Tohma and Y. Kita, Top. Curr. Chem., 2003, 224, 209. CrossRef
3. For selected recent summarizations of hypervalent iodine chemistry, see: (a) T. Wirth, M. Ochiai, A. Varvoglis, V. V. Zhdankin, G. F. Koser, H. Tohma, and Y. Kita, Hypervalent Iodine Chemistry Modern Developments in Organic Synthesis, ed. by T. Wirth, Springer-Verlag, Berlin (2003); CrossRef (b) V. V. Zhdankin and P. J. Stang, Chem. Rev., 2008, 108, 5299; CrossRef (c) T. Dohi and Y. Kita, Chem. Commun., 2009, 2073; CrossRef (d) L. F. Silva, Jr. and B. Olofsson, Nat. Prod. Rep., 2011, 28, 1722; CrossRef (e) D. Fernandez Gonzalez, F. Benfatti, and J. Waser, ChemCatChem, 2012, 4, 955; CrossRef (f) M. S. Yusubov and V. V. Zhdankin, Curr. Org. Synth., 2012, 9, 247; CrossRef (g) A. Parra and S. Reboredo, Chem. Eur. J., 2013, 19, 17244. CrossRef
4. (a) Y. Tamura, T. Yakura, J. Haruta, and Y. Kita, J. Org. Chem., 1987, 52, 3927; CrossRef (b) Y. Tamura, T. Yakura, H. Tohma, K. Kikuchi, and Y. Kita, Synthesis, 1989, 126; CrossRef (c) Y. Kita, T. Yakura, H. Tohma, K. Kikuchi, and Y. Tamura, Tetrahedron Lett., 1989, 30, 1119; CrossRef (d) Y. Kita, H. Tohma, K. Kikuchi, M. Inagaki, and T. Yakura, J. Org. Chem., 1991, 56, 435; CrossRef Applications of phenolic oxidations in our laboratory: (e) Y. Kita, H. Tohma, M. Inagaki, K. Hatanaka, and T. Yakura, J. Am. Chem. Soc., 1992, 114, 2175; CrossRef (f) Y. Kita, T. Takada, M. Gyoten, H. Tohma, M. H. Zenk, and J. Eichhorn, J. Org. Chem., 1996, 61, 5857; CrossRef (g) Y. Kita, M. Arisawa, M. Gyoten, M. Nakajima, R. Hamada, H. Tohma, and T. Takada, J. Org. Chem., 1998, 63, 6625; CrossRef (h) Y. Kita, M. Egi, T. Takada, and H. Tohma, Synthesis, 1999, 885; CrossRef (i) Y. Kita, M. Egi, and H. Tohma, Chem. Commun., 1999, 143; CrossRef (j) H. Tohma, Y. Harayama, M. Hashizume, M. Iwata, Y. Kiyono, M. Egi, and Y. Kita, J. Am. Chem. Soc., 2003, 125, 11235; CrossRef (k) H. Hamamoto, Y. Shiozaki, H. Nambu, K. Hata, H. Tohma, and Y. Kita, Chem. Eur. J., 2004, 10, 4977; CrossRef (l) Y. Wada, K. Otani, N. Endo, Y. Harayama, D. Kamimura, M. Yoshida, H. Fujioka, and Y. Kita, Org. Lett., 2009, 11, 4048; CrossRef For recent investigations, see: (m) T. Dohi, A. Maruyama, M. Yoshimura, K. Morimoto, H. Tohma, and Y. Kita, Angew. Chem. Int. Ed., 2005, 44, 6193; CrossRef (n) T. Dohi, Y. Minamitsuji, A. Maruyama, S. Hirose, and Y. Kita, Org. Lett., 2008, 10, 3559; CrossRef (o) Y. Minamitsuji, D. Kato, H. Fujioka, T. Dohi, and Y. Kita, Aust. J. Chem., 2009, 62, 648. CrossRef
5. (a) K. Morimoto, T. Dohi, and Y. Kita, Eur. J. Org. Chem., 2013, 1659; CrossRef (b) K. Morimoto, K. Sakamoto, Y. Ohnishi, T. Miyamoto, M. Ito, T. Dohi, and Y. Kita, Chem. Eur. J., 2013, 19, 8726. CrossRef
6. (a) S. Quideau, M. A. Looney, and L. Pouységu, Org. Lett., 1999, 1, 1651; CrossRef (b) A. Ozanne-Beaudenon and S. Quideau, Angew. Chem. Int. Ed., 2005, 44, 7065. CrossRef
7. (a) C. Sabot, B. Commare, M.-A. Duceppe, S. Nahi, K. C. Guerard, and S. Canesi, Synlett, 2008, 3226; (b) C. Sabot, K. C. Guerard, and S. Canesi, Chem. Commun., 2009, 2941; CrossRef (c) K. C. Guerard, C. Sabot, L. Racicot, and S. Canesi, J. Org. Chem., 2009, 74, 2039. CrossRef
8. (a) D. Berard, M.-A. Giroux, L. Racicot, C. Sabot, and S. Canesi, Tetrahedron, 2008, 64, 7537; CrossRef (b) C. Sabot, D. Berard, and S. Canesi, Org. Lett., 2008, 10, 4629; CrossRef (c) G. Jacquemot, M.-A. Menard, C. L'Homme, and S. Canesi, Chem. Sci., 2013, 4, 1287. CrossRef
9. (a) S. Wang, B. D. Gates, and J. S. Swenton, J. Org. Chem., 1991, 56, 1979; CrossRef (b) B. D. Gates, P. Dalidowicz, A. Tebben, S. Wang, and J. S. Swenton, J. Org. Chem., 1992, 57, 2135; CrossRef A recent application of intramolecular version: (c) D.-Q. Sun, Q.-W. Zhao, and C.-Z. Li, Org. Lett., 2011, 13, 5302. For other recent studies and utilizations of these types of reactions, see ref. 8.
10. H. Tohma, H. Watanabe, S. Takizawa, T. Maegawa, and Y. Kita, Heterocycles, 1999, 51, 1785. CrossRef
11. (a) T. Dohi, A. Maruyama, N. Takenage, K. Senami, Y. Minamitsuji, H. Fujioka, S. Cämmerer, and Y. Kita, Angew. Chem. Int. Ed., 2008, 47, 3787; CrossRef (b) T. Dohi, D. Kato, R. Hyodo, D. Yamashita, M. Shiro, and Y. Kita, Angew. Chem. Int. Ed., 2011, 50, 3784; CrossRef (c) T. Dohi, N. Takenaga, K. Fukushima, T. Uchiyama, D. Kato, M. Shiro, H. Fujioka, and Y. Kita, Chem. Commun., 2010, 46, 7697; CrossRef d) T. Dohi, T. Nakae, Y. Ishikado, D. Kato, and Y. Kita, Org. Biomol. Chem., 2011, 9, 6899; CrossRef (e) T. Dohi, N. Takenaga, T. Nakae, Y. Toyoda, M. Yamasaki, M. Shiro, H. Fujioka, A. Maruyama, and Y. Kita, J. Am. Chem. Soc., 2013, 135, 4558; CrossRef (f) M. Ito, H. Kubo, I. Itani, K. Morimoto, T. Dohi, and Y. Kita, J. Am. Chem. Soc., 2013, 135, 14078. CrossRef
12. (a) N. Takenaga, T. Uchiyama, D. Kato, H. Fujioka, T. Dohi, and Y. Kita, Heterocycles, 2011, 82, 1327; CrossRef (b) T. Dohi, T. Uchiyama, D. Yamashita, N. Washimi, and Y. Kita, Tetrahedron Lett., 2011, 52, 2212; CrossRef (c) T. Dohi, N. Nakae, N. Takenaga, T. Uchiyama, K. Fukushima, H. Fujioka, and Y. Kita, Synthesis, 2012, 44, 1183. CrossRef
13. (a) N. W. Alcock and T. C. Waddington, J. Chem. Soc., 1963, 4103; CrossRef (b) J. Gallos, A. Varvoglis, and N. W. Alcock, J. Chem. Soc., Perkin Trans. 1, 1985, 757. CrossRef
14. Reviews of syntheses and utilities of dihydrobenzofurans: (a) F. Bertolini and M. Pineschi, Org. Prep. Proced. Int., 2009, 41, 385; CrossRef (b) T. D. Sheppard, J. Chem. Res., 2011, 35, 377; CrossRef some recent reports: (c) U. A. Kshirsagar, C. Regev, R. Parnes, and D. Pappo, Org. Lett., 2013, 15, 3174; CrossRef (d) H. Adams, N. J. Gilmore, S. Jones, M. P. Muldowney, S. H. von Reuss, and R. Vemula, Org. Lett., 2008, 10, 1457; CrossRef (e) Z. Huang, L. Jin, Y. Feng, P. Peng, H. Yi, and A. Lei, Angew. Chem. Int. Ed., 2013, 52, 7151; CrossRef (f) K. Chiba, M. Fukuda, S. Kim, Y. Kitano, and M. Tada, J. Org. Chem., 1999, 64, 7654. CrossRef
15. (a) Y. Kita, H. Tohma, K. Hatanaka, T. Takada, S. Fujita, S. Mitoh, H. Sakurai, and S. Oka, J. Am. Chem. Soc., 1994, 116, 3684; CrossRef Accounts: (b) Y. Kita, T. Takada, and H. Tohma, Pure Appl. Chem., 1996, 68, 627; CrossRef (c) T. Dohi, M. Ito, N. Yamaoka, K. Morimoto, H. Fujioka, and Y. Kita, Tetrahedron, 2009, 65, 10797; CrossRef For the high sensitivities of naphthalenes toward the SET-oxidations, see: (d) T. Dohi, M. Ito, K. Morimoto, M. Iwata, and Y. Kita, Angew. Chem. Int. Ed., 2008, 47, 1301. CrossRef
16. These fluoroalcohols are useful in many hypervalent iodine-mediated phenolic oxidations. For accounts and reviews discussing their property and utility, see: (a) L. Eberson, M. P. Hartshorn, O. Persson, and F. Radner, Chem. Commun., 1996, 2105; CrossRef (b) J.-P. Bégué, D. Bonnet-delpon, and B. Crousse, Synlett, 2004, 18; (c) T. Dohi, N. Yamaoka, and Y. Kita, Tetrahedoron, 2010, 66, 5775. See, also ref. 15b.
17. (a) P. Ruiz-Sanchis, S. A. Savina, F. Albericio, and M. Álvarez, Chem. Eur. J., 2011, 17, 1388; CrossRef (b) D. Zhang, H. Song, and Y. Qin, Acc. Chem. Res., 2011, 44, 447; CrossRef (c) M. Ishikura, K. Yamada, and T. Abe, Nat. Prod. Rep., 2010, 27, 1630; CrossRef (d) J. Kim and M. Movassaghi, Chem. Soc. Rev., 2009, 38, 3035; CrossRef (e) A. Steven and L. E. Overman, Angew. Chem. Int. Ed., 2007, 46, 5488; CrossRef (f) D. Crich and A. Banerjee, Acc. Chem. Res., 2007, 40, 151. CrossRef
18. (a) B. G. Katzung, S. B. Masters, and A. J. Trevor, Basic and Clinical Pharmacology, 11th ed., McGraw Hill, 2009; (b) B. Witkop, Heterocycles, 1998, 49, 9. CrossRef
19. R. N. Nair, P. J. Lee, A. L. Rheingold, and D. B. Grotjahn, Chem. Eur. J., 2010, 16, 7992. CrossRef