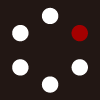
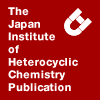
HETEROCYCLES
An International Journal for Reviews and Communications in Heterocyclic ChemistryWeb Edition ISSN: 1881-0942
Published online by The Japan Institute of Heterocyclic Chemistry
e-Journal
Full Text HTML
Received, 26th June, 2014, Accepted, 29th July, 2014, Published online, 19th August, 2014.
DOI: 10.3987/COM-14-S(K)43
■ Synthesis of Bicyclic Dioxetanes Bearing a Hydroxyphenanthrene or Hydroxy[4]helicene Moiety and Their Base-Induced Chemiluminescent Decomposition
Yohei Koyama, Nobuko Watanabe, Hisako K. Ijuin, and Masakatsu Matsumoto*
Department of Chemistry, Faculty of Science, Kanagawa University, 2946 Tsuchiya, Hiratsuka 259-1293, Japan
Abstract
Six bicyclic dioxetanes bearing a 1-hydroxyphenanthren-3-yl 2-iv, 3-hydroxyphenanthren-1-yl 2-ov, 4-hydroxyphenanthren-2-yl 2-oh, 2-hydroxyphenanthren-4-yl 2-ih, 4-hydroxy[4]helicen-2-yl 3-iv and 2-hydroxy[4]helicen-4-yl group 3-ov were synthesized and their base-induced chemiluminescent decomposition was investigated in a TBAF (tetrabutylammonium fluoride) / MeCN system. For dioxetanes in the iv-series 2-iv and 3-iv including α-naphthol-analog 17a and those in the ov-series 2-ov and 3-ov including β-naphthol-analog 17b, we investigated how the chemiluminescence properties changed with an increase in the number of fused benzene rings of a hydroxyarene moiety attached to a dioxetane ring. The results showed that a) maximum wavelength of chemiluminescence λmaxCL tended to shift to a longer wavelength region as the number of fused benzene rings increased, b) the kCTID values for the iv-series were >1000 times larger than those for the ov-series regardless of the number of fused benzene rings, and c) a dioxetane in the iv-series tended to have a higher singlet-chemiexcitation efficiency ΦS than the corresponding dioxetane in the ov-series. This tendency could be explained by the “syn/anti” rotational isomerism of an aromatic electron donor, where an anti-rotamer gives ΦS far more effectively than a syn-rotamer.INTRODUCTION
A dioxetane bearing an aromatic electron donor such as oxidoaryl anion undergoes intramolecular charge-transfer-induced decomposition (CTID) with an accompanying emission of bright light.1−6 This phenomenon has received considerable attention from the viewpoints of mechanistic interest related to bioluminescence and its potential for application to clinical and biological analysis.7,8 Thus, intensive studies have been conducted, and have shown that an aromatic electron donor significantly affects the chemiluminescence properties such as the chemiluminescence spectrum, chemiluminescence efficiency and the rate of decomposition for CTID-active dioxetanes. Such effects are mainly due to the structure of the π-electron system and the bulkiness of the aromatic electron donor.
On the other hand, the chirality of an aromatic electron donor such as a biaryl due to atropisomerism leads to unique chemiluminescence for a CTID-active dioxetane. For instance, optically active bicyclic dioxetanes 1 bearing a chiral binaphthyl moiety have very recently been found to show chiral-induced chemiluminescence in an optically anisotropic microenvironment (Figure 1).9,10 In addition to this phenomenon, it would be interesting to determine whether or not CTID of optically active dioxetanes shows the emission of polarized light. For observing such an unprecedented phenomenon, an important preliminary subject may be to design and synthesize dioxetanes bearing a helicene-type electron donor that could be expected to exhibit a large specific rotation due to its large helicity (Figure 1).
A promising candidate for such a CTID-active dioxetane would be one bearing a hydroxyhelicene moiety. However, there are too many substitution patterns when both a hydroxy group as a trigger and a dioxetane ring are introduced to an ortho-fused polyacene ring: there are 66 patterns for a [4]helicene (benzo[c]phenanthrene) and 91 for [5]helicene! Furthermore, little is currently known about the synthetic feasibility and chemiluminescence properties of a dioxetane bearing a helicene moiety. In light of the chemiluminescence properties for the CTID of dioxetanes bearing a hydroxynaphthyl group,11 we decided to fundamentally investigate the CTID of racemic bicyclic dioxetanes bearing a phenanthrene moiety in which both a hydroxy group and a dioxetane ring lie in an “odd” relationship on the A-ring.11,12 The thus-realized dioxetanes were 2-iv, 2-ov, 2-oh and 2-ih, as shown in Figure 2.13 Based on an evaluation with respect to the chemiluminescence properties, thermal stability, and synthetic feasibility of 2, two selected dioxetanes bearing a hydroxyl[4]helicene moiety 3-iv and 3-ov were further synthesized and their CTID was investigated.
RESULTS AND DISCUSSION
Synthesis of bicyclic dioxetanes bearing a hydroxyphenanthrene or hydroxy[4]helicene moiety
Bicyclic dioxetanes 2 bearing a hydroxyphenanthrene moiety were synthesized by singlet oxygenation of the corresponding dihydrofurans 4. The key step in the synthesis of precursors 4 was the construction of di-substituted phenanthrene rings. Thus, we applied the cyclization of an o-halogeno-Z-stilbene using Bu3SnH.14 The synthetic sequence leading to precursor 4-iv for dioxetane 2-iv is described here as a representative example (Scheme 1). First, we conducted a Wittig reaction of dihydrofuran 5a bearing a 4-formyl-3-methoxyphenyl group with 2-iodobenzyltriphenylphosphorane prepared fromphosphonium bromide 6 to give dihydrofuran 7a bearing a 3-methoxyphenyl moiety substituted with a Z-(2-iodophenyl)ethenyl group at the 4-position in 96% yield along with a small amount of its E-isomer. When 7a and Bu3SnH were heated in the presence of AIBN (azobisisobutyronitrile) in toluene, the desired 1-methoxyphenanthren-3-yl-substituted dihydrofuran 8-iv was produced in 85% isolated yield. Demethylation of a methoxy group in 8-iv was attained by heating with sodium thiomethylate in DMF to give precursor 4-iv in 99% yield.
As shown in Scheme 1, Z-stilbene-type compounds 7b and 7c were also prepared by the Wittig reaction of the corresponding aldehydes 5b and 5c. Cyclization of 7b as for 7a gave 3-methoxyphenanthrene 8-ov in 86% yield, while the similar cyclization of 7c gave 4-methoxyphenanthrene 8-oh and its 2-methoxy-isomer 8-ih in respective yields of 58% and 31%. These methoxyphenanthryl-substituted dihydrofurans 8-ov, 8-oh and 8-ih were demethylated as in the case of 8-iv to give the corresponding hydroxyphenanthryl-substituted dihydrofurans 4-ov, 4-oh and 4-ih in yields of 87-98%.
When dihydrofuran 4-iv was irradiated with a Na-lamp in the presence of a catalytic amount of tetraphenylporphin (TPP) in CH2Cl2 under an O2 atmosphere at 0 °C, 1,2-addition of singlet oxygen to 4-iv effectively proceeded to give thermally stable dioxetane 2-iv in 78% isolated yield. Similar singlet oxygenation of precursors 4-ov and 4-oh smoothly took place to give thermally stable dioxetanes 2-ov and 2-oh in respective yields of 96% and 68%. On the other hand, singlet oxygenation of 4-ih proceeded to mainly give keto ester 9-ih, which was produced from thermally unstable dioxetane 2-ih: all efforts to isolate pure 2-ih were unsuccessful, so that we could obtain only a mixture of 2-ih and 9-ih. The structures of dioxetanes 2-iv, 2-ov and 2-oh were determined by 1H NMR, 13C NMR, IR, Mass and HRMass spectral analyses: the structure of 2-ih was determined by 1H NMR. All dioxetanes 2-iv, 2-ov and 2-oh selectively gave the corresponding keto esters 9-iv, 9-ov and 9-oh when heated in p-xylene (Figure 3).
Synthetic feasibility for dioxetanes 2-iv, 2-ov, 2-oh, and 2-ih suggested that the design of dioxetanes bearing a hydroxyhelicene moiety appeared to be promising for the development of dioxetanes such as 2-iv and 2-ov. Thus, two selected dioxetanes bearing a hydroxy[4]helicene moiety 3-iv and 3-ov were synthesized according to a strategy similar to that for dioxetanes 2. The precursor dihydrofurans 10-iv and 10-ov bearing a hydroxy[4]helicene moiety were synthesized starting from dihydrofurans 5a and 5b, respectively (Scheme 2). First, dihydrofurans 5a and 5b were reacted with a phosphorane prepared fromphosphonium bromide 11 to give the corresponding Z-diarylethenes 12a and 12b in good yields. Cyclization of 12a and 12b was carried out as in the case of 7 to give the corresponding methoxy[4]helicene-substituted dihydrofurans 13-iv and 13-ov in respective yields of 45 and 62%. These
methoxyaryl-substituted dihydrofurans 13-iv and 13-ov were demethylated as in the case of 8-iv to effectively give the precursor dihydrofurans 10-iv and 10-ov, respectively. Finally, singlet oxygenation of 10-iv and 10-ov was carried out at 0 °C to give dioxetanes 3-iv and 3-ov in respective yields of 60 and 86%. These dioxetanes were thermally stable, but selectively decomposed to the corresponding keto esters 14-iv and 14-ov under heating in p-xylene. Dioxetanes 3-iv and 3-ov gave satisfactory 1H NMR, 13C NMR, Mass, and HRMass spectral data. Furthermore, X-ray single-crystallographic analysis was successfully performed for both dioxetanes 3-iv and 3-ov. Figure 4 shows their ORTEP views, from which we can infer that the [4]helicene ring is skewed by about 37°.
Base-induced chemiluminescent decomposition of bicyclic dioxetanes bearing a hydroxyphenanthrene or hydroxy[4]helicene moiety
When a solution of 2-iv in acetonitrile was added to a solution of tetrabutylammonium fluoride (TBAF, large excess) in acetonitrile at 25 ºC, 2-iv decomposed according to pseudo-first order kinetics independent of the TBAF concentration with an accompanying emission of yellow light, the spectrum of which is shown in Figure 6. The chemiluminescence properties of 2-iv were as follows: maximum wavelength λmaxCL = 506 nm, chemiluminescence efficiency ΦCL = 5.2 x 10-2,15,16 rate of CTID kCTID = 2.5 s-1, and half-life t1/2CTID = 0.28 s (Table 1). The spent reaction mixture exclusively gave keto ester 9-iv after careful neutralization. Authentic oxido anion 15-iv generated from 9-iv in TBAF/acetonitrile gave fluorescence, the spectrum of which coincided with the chemiluminescence spectrum of 2-iv. These results indicate that CTID of 2-iv proceeded through an intermediary dioxetane 16-iv to give anionic keto ester 15-iv accompanied by the emission of light (Scheme 3). Thus, based on the fluorescence efficiency (Φfl) of 15-iv, the chemiexcitation efficiency (ΦS = ΦCL/Φfl) was estimated to be 0.10 (Table 1).
Under similar treatment with TBAF in acetonitrile, 2-ov, 2-oh, and 2-ih showed chemiluminescence, the spectra and properties of which are shown in Figure 6 and Table 1: 2-ih was used as a mixture that included a large amount (ca. 30%) of 9-ih. The chemiluminescence properties for dioxetanes 2 were compared to those for dioxetanes bearing a rather simple hydroxyaryl group, i.e., a 1-hydroxynaphthalen-3-yl 17a or 3-hydroxynaphthalen-1-yl group 17b, together with those for dioxetane 18 bearing a 3-hydroxyphenyl group (Figures 5 and 6 and Table 1): 17a was synthesized according to the procedure for 17b.9b The data of Table 1 show that a) the ΦCL values for all three of the hydroxyphenanthryl-substituted dioxetanes 2-iv, 2-ov, and 2-oh were higher than or comparable to that for hydroxynaphthyl-substituted dioxetane 17b though lower than that for 17a, and b) the kCTID values for dioxetanes bearing a β-naphthol-type arene moiety, i.e., 17b and 2-ov, were far lower than those for dioxetanes bearing an α-naphthol-type arene moiety, i.e., 17a, 2-iv and 2-oh.
When dioxetane 3-iv was treated with TBAF in acetonitrile as with 2, 3-iv decomposed to give a flash of yellow light with λmaxCL = 559 nm , the spectrum of which is shown in Figure 6 [A] together with those of 2-iv, 2-ih, 17a and 18. On the other hand, the TBAF-induced decomposition of 3-ov showed a glowing light with λmaxCL = 530 nm, the spectrum of which is shown in Figure 6 [B] together with those of 2-oh, 2-ov, 17b and 18. The chemiluminescence properties for 3-iv and 3-ov are summarized in Table 1, which shows that their ΦCL values were somewhat lower than that for 2-ov. Thus, we attempted to
understand how λmaxCL, kCTID and ΦCL changed with a change in the number of fused benzene rings from phenol to hydroxy[4]helicene for two series, i.e., the iv-series and ov-series, of hydroxyaryl-substituted dioxetanes, 18 → 17 → 2 → 3.
Table 1 shows that λmaxCL tended to shift to a longer wavelength region for both the iv-series and the ov-series as the number of fused benzene rings increased, with the exception of 2-ov. This tendency may be due to the expansion of the π-conjugation system from oxidophenyl anion → oxidonaphthyl anion → oxidophenanthryl anion and finally to oxido[4]helicenyl anion. On the other hand, the kCTID values for the iv-series were >1000 times larger than those for the ov-series regardless of the number of fused benzene rings. The significant difference in kCTID values between the iv-series and ov-series is presumably attributed to the difference in the ease of oxidation of oxidoarene anions, which act as an electron donor for CTID. In fact, the anion of α-naphthol, corresponding to 17a, has been reported to possess a formal oxidation potential (E = -498 mV vs Ag/Ag+ in DMSO) that is considerably lower than that of an anion of β-naphthol, corresponding to 17b (E = -369 mV).18 This may also be the case for 2 and 3: all of the dioxetanes in the iv-series have an α-naphthol-type arene moiety, while those in the ov-series have a β-naphthol-type arene moiety.
Table 1 further shows that the ΦS value for a dioxetane bearing an α-naphthol-type arene (Table 1) tended to be higher than that for the corresponding dioxetane bearing a β-naphthol-type arene: 17a >> 17b, 2-oh >> 2-iv > 2-ov, and 3-iv > 3-ov. This tendency can be explained by the “syn/anti” rotational isomerism of an aromatic electron donor, in which an anti-rotamer gives ΦS far more effectively than a syn-rotamer (Scheme 4).19,20
A syn-rotamer ,typically shown in Figure 4, is likely to be more stable than an anti-rotamer for dioxetanes in the ov-series because of steric hindrance by a peri-hydrogen of an aromatic electron donor (Scheme 5). Thus, we carried out an MO calculation (Gaussian 03, HF/6-31G) to estimate the energy difference ΔEanti – syn between the syn- and anti-rotamers and the barriers to rotation ΔErotation from the most stable rotamer (syn or anti) to the other stable rotamer (anti or syn) (Table 2). The results show that a) the syn-rotamer is more stable than the anti-rotamer for the ov-series, while the anti-rotamer is more stable for the iv-series, and b) ΔErotation values for the ov-series tend to be higher than those for the iv-series. Thus, we can see that, for a dioxetane in the iv-series, the anti-rotamer is presumably predominant compared to the syn-rotamer, while a dioxetane in the ov-series shows the opposite tendency. Hence, a dioxetane in the iv-series would give a singlet-excited emitter more effectively than the corresponding dioxetane in the ov-series under these reaction conditions.
CONCLUSION
Four bicyclic dioxetanes bearing a phenanthrene moiety possessing a hydroxy group in the A-ring 2-iv, 2-ov, 2-oh, and 2-ih were synthesized. Except for 2-ih, these dioxetanes were thermally stable and underwent TBAF-induced decomposition with the accompanying emission of bright light. As an extension, we synthesized two dioxetanes 3-iv and 3-ov bearing a 4-hydroxy[4]helicen-2-yl or 2-hydroxy[4]helicen-4-yl group and found that their CTID effectively gave light. Thus, we investigated how the chemiluminescence properties of the iv-series and ov-series of dioxetanes changed with an increase in the number of fused benzene rings of a hydroxyarene moiety attached to a dioxetane ring. The results showed that a) λmaxCL tended to shift to a longer wave-length region for both the iv-series and the ov-series as the number of fused benzene rings increased, b) the kCTID values for the iv-series were >1000 times larger than those for the ov-series regardless of the number of fused benzene rings, and c) the ΦS value for a dioxetane bearing an α-naphthol-type arene (iv-series) tended to be higher than that for the corresponding dioxetane bearing a β-naphthol-type arene (ov-series): 17a >> 17b, 2-oh >> 2-iv > 2-ov, and 3-iv > 3-ov. This tendency could be explained by the “syn/anti” rotational isomerism of an aromatic electron donor, in which an anti-rotamer gives ΦS far more effectively than a syn-rotamer.
EXPERIMENTAL
General
Melting points were uncorrected. IR spectra were taken on a FT/IR infrared spectrometer. 1H and 13C NMR spectra were recorded on a 300 MHz, 400 MHz and 500 MHz spectrometers. Mass spectra were obtained using double-focusing mass spectrometers and an ESI-TOF mass spectrometer. Column chromatography was carried out using silica gel.
Synthesis of 4-tert-butyl-5-{4-[Z-2-(2-iodophenyl)ethenyl]-3-methoxyphenyl}-3,3-dimethyl-2,3-dihydrofuran (7a): typical procedure.
Potassium tert-butoxide (1.82 g, 16.3 mmol) was added to a solution of 2-iodobenzyltriphenylphosphonium bromide (6) (7.43 g, 13.3 mmol) in dry THF (100 mL) under a nitrogen atmosphere at 0 °C and stirred for 30 min. To the solution was added dropwise 4-tert-butyl-5-(4-formyl-3-methoxyphenyl)-3,3-dimethyl-2,3-dihydrofuran (5a) (3.56 g, 12.4 mmol) in dry THF (90 mL) over 10 min at room temperature, and the mixture was stirred for 3 h. The reaction mixture was poured into H2O and extracted with Et2O. The organic layer was washed with sat. aq. NaCl, dried over anhydrous MgSO4 and concentrated in vacuo. The residue was chromatographed on silica gel with AcOEt−hexane (1:4) to give 7a (5.80 g, 11.9 mmol, 96%).
7a: colorless oil. 1H NMR (400 MHz, CDCl3): δΗ 1.03 (s, 9H), 1.31 (s, 6H), 3.81 (s, 3H), 3.86 (s, 2H), 6.56 (d, J = 12.2 Hz, 1H), 6.62 (dd, J = 7.7 and 1.4 Hz, 1H), 6.76 (s with fine coupling, 1H), 6.78 (d, J = 12.2 Hz, 1H), 6.82−6.88 (m, 1H), 6.87 (d, J = 7.7 Hz, 1H), 6.99−7.08 (m, 2H), 7.84 (dd, J = 7.7 and 1.0 Hz, 1H) ppm. 13C NMR (125 MHz, CDCl3): δC 27.4, 32.4, 32.4, 47.2, 55.5, 83.1, 99.8, 112.1, 122.0, 125.1, 125.8, 126.3, 127.7, 128.4, 129.6, 130.3, 134.1, 136.5, 138.8, 141.6, 149.7, 156.7 ppm. IR (liquid film): ν̃ 3050, 2956, 2865, 1650, 1601, 1582, 1559 cm-1. Mass (m/z, %): 488 (M+, 31), 474 (24), 473 (100), 363 (19), 345 (30), 215 (16), 165 (35). HRMS (ESI): 489.1303, calcd for C25H30IO2 [M+H+] 489.1291; 511.1106, calcd for C25H29IO2Na [M+Na+] 511.1110; 527.0849, calcd for C25H29IO2K [M+K+] 527.0849.
7b: 57% yield. colorless columns, mp 76.5−77.0 °C (from AcOEt−hexane). 1H NMR (500 MHz, CDCl3): δΗ 1.10 (s, 9H), 1.37 (s, 6H), 3.76 (s, 3H), 3.91 (s, 2H), 6.44 (d, J = 12.1 Hz, 1H), 6.54 (dd, J = 8.6 and 2.8 Hz, 1H), 6.65 (d, J = 12.1 Hz, 1H), 6.77 (d, J = 2.8 Hz, 1H), 6.86−6.90 (m, 1H), 6.89 (d, J = 8.6 Hz, 1H), 7.10 (ddd, J = 7.6, 7.3 and 0.9 Hz, 1H), 7.16 (dd, J = 7.6 and 1.6 Hz, 1H), 7.87 (dd, J = 8.0 and 0.9 Hz, 1H) ppm. 13C NMR (125 MHz, CDCl3): δC 27.2, 32.1, 32.6, 47.2, 55.2, 83.2, 100.1, 113.7, 115.6, 126.6, 127.8, 128.1, 128.4, 128.9, 130.2, 130.3, 132.4, 137.2, 138.9, 141.8, 148.0, 158.3 ppm. IR (liquid film): ν̃ 2963, 2860, 1604, 1564, 1461, 1307 cm-1. Mass (m/z, %): 488 (M+, 38), 473 (28), 431 (17) 256 (7). HRMS (ESI): 511.1110, calcd for C25H29IO2Na [M+Na+] 511.1110; 527.0861, calcd for C25H29IO2K [M+K+] 527.0849.
7c: 58% yield. colorless oil. 1H NMR (400 MHz, CDCl3): δH 0.98 (s, 9H), 1.28 (s, 6H), 3.59 (s, 3H), 3.82 (s, 2H), 6.52 (d, J = 12.0 Hz, 1H), 6.57 (dd, J = 2.4 and 1.3 Hz, 1H), 6.61 (d, J = 12.0 Hz, 1H), 6.64 (dd, J = 2.4 and 1.3 Hz, 1H), 6.67 (s with fine coupling, 1H), 6.87−6.92 (m, 1H), 7.11−7.18 (m, 2H), 7.85−7.88 (m, 1H) ppm. 13C NMR (125 MHz, CDCl3): δC 27.3, 32.3, 32.5, 47.1, 55.0, 83.0, 99.5, 113.7, 114.8, 123.4, 125.5, 128.0, 128.6, 130.4, 130.8, 134.0, 137.1, 137.3, 139.0, 141.6, 149.4, 158.8 ppm. IR (liquid film): ν̃ 2955, 2866, 1653, 1585, 1464 cm-1. Mass (m/z, %): 488 (M+, 28), 474 (25), 473 (100). HRMS (ESI): 489.1292, calcd for C25H30IO2 [M+H+] 489.1291; 511.1104, calcd for C25H29IO2Na [M+Na+] 511.1110.
12a: 84% yield. colorless columns, mp 101.0−102.0 °C (from AcOEt−hexane). 1H NMR (400 MHz, CDCl3): δΗ 1.03 (s, 9H), 1.31 (s, 6H), 3.84 (s, 3H), 3.86 (s, 2H), 6.57 (dd, J = 7.8 and 1.4 Hz, 1H), 6.79 (d, J = 1.4 Hz, 1H), 6.90 (d, J = 7.8 Hz, 1H), 6.93 (s, 2H), 7.16 (d, J = 8.5 Hz, 1H), 7.45 (d, J = 8.5 Hz, 1H), 7.48 (dd with fine coupling, J = 8.0 and 6.9 Hz, 1H), 7.57 (dd with fine coupling, J = 8.5 and 6.9 Hz, 1H), 7.72 (d with fine coupling, J = 8.0 Hz, 1H), 8.34 (d with fine coupling, J = 8.5 Hz, 1H) ppm. 13C NMR (125 MHz, CDCl3): δC 27.4, 32.4, 32.4, 47.1, 55.5, 83.1, 112.0, 121.9, 123.7, 125.2, 125.8, 126.3, 126.7, 126.8, 127.1, 127.1, 128.0, 128.2, 129.9, 130.7, 132.4, 133.5, 136.2, 136.7, 149.6, 156.7 ppm. IR (KBr): ν̃ 2954, 2925, 2855, 1650, 1604, 1463 cm-1. Mass (m/z, %): 492 (M++2, 36), 490 (M+, 37), 478 (28), 477 (100), 475 (98), 395 (32), 367 (35), 365 (35), 340 (48), 215 (49). HRMS (ESI): 513.1399, calcd for C29H3179BrO2Na [M+Na+] 513.1405; 515.1387, calcd for C29H3181BrO2Na [M+Na+] 515.1385.
12b: 72% yield. colorless columns, mp 129.0−130.0 °C (from Et2O−hexane). 1H NMR (500 MHz, CDCl3): δΗ 1.12 (s, 9H), 1.39 (s, 6H), 3.75 (s, 3H), 3.93 (s, 2H), 6.49 (dd, J = 8.6 and 2.8 Hz, 1H), 6.78 (d, J = 12.1 Hz, 1H), 6.79 (d, J = 2.8 Hz, 1H), 6.81 (d, J = 12.1 Hz, 1H), 6.92 (d, J = 8.6 Hz, 1H), 7.25 (d, J = 8.6 Hz, 1H), 7.49 (dd with fine coupling, J = 7.9 and 6.9 Hz, 1H), 7.53 (d, J = 8.6 Hz, 1H), 7.57 (dd with fine coupling, J = 8.6 and 6.9 Hz, 1H), 7.74 (d, J = 7.9 Hz, 1H), 8.34 (d, J = 8.6 Hz, 1H) ppm. 13C NMR (125 MHz, CDCl3): δC 27.3, 32.2, 32.6, 47.2, 55.2, 83.3, 113.7, 115.7, 123.8, 128.3, 126.3, 126.6, 127.0, 127.1, 127.2, 128.0, 128.3, 129.2, 129.4, 130.7, 132.5, 133.5, 136.6, 137.3, 148.0, 158.4 ppm. IR (KBr): ν̃ 2956, 2863, 1602, 1487, 1049 cm-1. Mass (m/z, %): 492 (M++2, 1), 490 (M+, 1), 252 (1), 221 (4), 215 (5), 57 (100). HRMS (ESI): not observed.
Synthesis of 4-tert-butyl-5-(1-methoxyphenanthren-3-yl)-3,3-dimethyl-2,3-dihydrofuran (8-iv): typical procedure. AIBN (177 mg, 1.08 mmol) and tributyltin hydride (3.40 mL, 12.6 mmol) were added to a solution of 7a (2.57 g, 5.26 mmol) in dry toluene (180 mL) under a nitrogen atmosphere at room temperature, and the solution was stirred at 90 °C for 10 h. The reaction mixture was concentrated in vacuo. The residue was crystallized from AcOEt−hexane to give 4-tert-butyl-5- (1-methoxyphenanthren-3-yl)-3,3-dimethyl-2,3-dihydrofuran (8-iv) (1.61 g, 4.47 mmol, 85%).
8-iv: colorless columns, mp 141.0−142.0 °C (from Et2O−hexane). 1H NMR (400 MHz, CDCl3): δΗ 1.11 (s, 9H), 1.42 (s, 6H), 3.98 (s, 2H), 4.05 (s, 3H), 6.93 (d , J = 1.0 Hz, 1H), 7.59 (dd with fine coupling, J = 7.7 and 6.9 Hz, 1H), 7.63 (dd with fine coupling, J = 8.2 and 6.9 Hz, 1H), 7.75 (d , J = 9.1 Hz, 1H), 7.89 (dd, J = 7.7 and 1.5 Hz, 1H), 8.21 (d, J = 9.1 Hz, 1H), 8.22 (s, 1H), 8.66 (d, J = 8.2 Hz, 1H) ppm. 13C NMR (125 MHz, CDCl3): δC 27.5, 32.5, 32.6, 47.3, 55.7, 83.1, 107.5, 116.7, 120.2, 122.8, 123.2, 126.0, 126.3, 126.4, 126.6, 128.5, 130.0, 130.9, 132.3, 134.4, 150.5, 155.5 ppm. IR (KBr): ν̃ 2959, 2866, 1654, 1611, 1599 cm-1. MASS (m/z, %): 360 (M+, 30), 346 (26), 345 (100), 289 (17), 235 (17), 57 (13). HRMS (ESI): 361.2168, calcd for C25H29O2 [M+H+] 361.2168; 383.1991, calcd for C25H28O2 Na [M+Na+] 383.1987; 415.2255, calcd for C26H32O3Na [M+MeOH+Na+]415.2249.
8-ov: 86% yield. colorless columns, mp 136.0−137.0 °C (from AcOEt−hexane). 1H NMR (500 MHz, CDCl3): δH 1.01 (s, 9H), 1.43 (s, 3H), 1.49 (s, 3H), 4.02 (qAB, J = 8.0 Hz, 2H), 4.02 (s, 3H), 7.21 (d, J = 2.4 Hz, 1H), 7.55−7.65 (m, 2H), 7.63 (d, J = 9.2 Hz, 1H), 7.81 (d, J = 9.2 Hz, 1H), 7.87 (dd, J = 7.6 and 1.1 Hz, 1H), 8.07 (d, J = 2.4 Hz, 1H), 8.60 (d, J = 8.0 Hz, 1H) ppm. 13C NMR (125 MHz, CDCl3): δC 27.3, 27.6, 32.0, 32.6, 47.3, 55.5, 83.4, 104.6, 118.8, 122.8, 124.3, 124.9, 125.8, 126.1, 126.6, 127.9, 128.5, 129.8, 131.8, 132.3, 135.8, 147.6, 157.5 ppm. IR (liquid film): ν̃ 2987, 2960, 2867, 1650, 1604, 1046 cm-1. Mass (m/z, %): 360 (M+, 9), 345 (8), 288 (12), 245 (12), 235 (11), 215 (16), 202 (17), 57(100). HRMS (ESI): 361.2170, calcd for C25H29O2 [M+H+] 361.2168; 383.1994, calcd for C25H28O2Na [M+Na+] 383.1987.
8-oh: 58% yield. colorless columns, mp 113.5−114.0 °C (from AcOEt−hexane). 1H NMR (300 MHz, CDCl3): δH 1.10 (s, 9H), 1.40 (s, 6H), 3.96 (s, 2H), 4.15 (s, 3H), 7.08 (d, J = 1.5 Hz, 1H), 7.48 (d, J = 1.5 Hz, 1H), 7.54−7.67 (m, 2H), 7.68 (d, J = 8.7 Hz, 1H), 7.74 (d, J = 8.7 Hz, 1H) 7.87 (dd, J = 7.6 and 1.8 Hz, 1H), 9.64 (d with fine coupling, J = 8.1 Hz, 1H) ppm. 13C NMR (125 MHz, CDCl3): δC 27.5, 32.5, 32.6, 47.3, 55.8, 83.3, 109.9, 120.4, 122.9, 126.0, 126.2, 126.4, 127.2, 128.1, 128.2, 128.6, 130.1, 132.9, 134.1, 134.3, 149.7, 158.4 ppm. IR (KBr): ν̃ 3064, 2984, 2951, 1655, 1601, 1567 cm-1. Mass (m/z, %): 360 (M+, 30), 346 (26), 345 (100), 289 (15), 235 (13). HRMS (ESI): 361.2179, calcd for C25H29O2 [M+H+] 361.2168; 383.1985, calcd for C25H28O2Na [M+Na+] 383.1987; 399.1771, calcd for C25H28O2K [M+K+] 399.1726.
8-ih: 31% yield. colorless columns, mp 162.5−163.0 °C (from AcOEt−hexane). 1H NMR (500 MHz, CDCl3): δH 0.97 (s, 9H), 1.44 (s, 3H), 1.52 (s, 3H), 3.92 (s, 3H), 4.10(qAB, J = 8.2 Hz, 2H) 7.18 (d, J = 2.9 Hz, 1H) 7.25 (d, J = 2.9 Hz, 1H), 7.50 (dd with fine coupling, J = 7.7 and 6.9 Hz, 1H), 7.54 (dd with fine coupling, J = 8.4 and 6.9 Hz, 1H) 7.62 (d, J = 8.8 Hz, 1H), 7.68 (d, J = 8.8 Hz, 1H), 7.82 (d with fine coupling, J = 7.7 Hz, 1H), 9.16 (d, J = 8.4 Hz, 1H) ppm. 13C NMR (125 MHz, CDCl3): δC 26.2, 27.9, 31.7, 32.7, 47.1, 55.4, 83.7, 110.0, 122.1, 123.4, 124.0, 125.4, 125.7, 126.4, 127.1, 127.9, 128.4, 130.5, 132.0, 134.3, 134.9, 151.1, 156.6 ppm. IR (KBr): ν̃ 3051, 2956, 2867, 1641, 1600, 1575, 1263, 1231, 1202 cm-1. Mass (m/z, %): 360 (M+, 28), 346 (25), 345 (100), 303 (13), 289 (14), 235 (12). HRMS (ESI): 361.2187, calcd for C25H29O2 [M+H+] 361.2168; 383.1989, calcd for C25H28O2Na [M+Na+] 383.1987; 399.1758, calcd for C25H28O2K [M+K+] 399.1726.
13-iv: 45% yield. colorless columns, mp 170.0−171.0 °C. (from CH2Cl2−hexane). 1H NMR (300 MHz, CDCl3): δH 1.15 (s, 9H), 1.41 (s, 6H), 3.96 (s, 2H), 4.10 (s, 3H), 6.94 (d, J = 1.0 Hz, 1H), 7.57−7.69 (m, 2H), 7.82 (d, J = 8.5 Hz, 1H), 7.83 (d, J = 8.8 Hz, 1H), 7.89 (d, J = 8.5 Hz, 1H), 8.00 ( dd, J = 7.5 and 1.8 Hz, 1H), 8.39 (d, J = 8.8 Hz, 1H), 8.64 ( s with fine coupling, 1H), 9.87 (d with fine coupling, J = 8.1 Hz, 1H) ppm. 13C NMR (125 MHz, CDCl3): δC 27.5, 32.6, 32.6, 47.3, 55.8, 83.2, 106.5, 120.8, 121.9, 124.7, 125.7, 125.9, 126.0, 126.4, 126.8, 127.2, 127.6, 128.1, 128.4, 130.4, 130.8, 131.4, 133.5, 134.1, 150.7, 155.4 ppm. IR (KBr): ν̃ 2958, 2866, 1652, 1601 cm-1. Mass (m/z, %): 410 (M+, 38), 396 (28), 395 (100), 339 (15), 285 (11), 242 (11), 214 (10). HRMS (ESI): 411.2331, calcd for C29H31O2 [M+H+] 411.2324; 433.2149, calcd for C29H30O2Na [M+Na+] 433.2144.
13-ov: 62% yield. colorless needls, mp 136.0−136.5 °C. (from CH2Cl2−hexane). 1H NMR (500 MHz, CDCl3): δH 1.05 (s, 9H), 1.45 (s, 3H), 1.51 (s, 3H), 4.01 (s, 3H), 4.04 (qAB, J = 8.1 Hz, 2H), 7.26 (d, J = 2.5 Hz, 1H), 7.60 (dd with fine coupling, J = 7.9 and 6.8 Hz, 1H), 7.66 (dd with fine coupling, J = 8.5 and 6.8 Hz, 1H) 7.72 (d, J = 8.6 Hz, 1H), 7.79 (d, J = 8.5 Hz, 1H), 7.87 (d, J = 8.5 Hz, 1H), 7.98 (d, J = 8.6 Hz, 1H), 8.01 (d with fine coupling, J = 7.9 Hz, 1H), 8.57 (d, J = 2.5 Hz, 1H), 9.16 (d, J = 8.5 Hz, 1H) ppm. 13C NMR (125 MHz, CDCl3): δC 27.3, 27.6, 32.0, 32.7, 47.4, 55.6, 83.4, 109.8, 118.6, 125.0 (x 2), 125.6, 125.9, 126.8, 126.9, 127.2, 127.5, 127.5, 128.0, 128.6, 130.5, 131.4, 131.7, 133.4, 135.5, 147.8, 157.2 ppm. IR (KBr): ν̃ 3052, 2956, 2862, 1648, 1601 cm-1. Mass (m/z, %): 410 (M+, 100), 396 (18), 395 (60), 380 (15), 365 (16), 339 (15). HRMS (ESI): 411.2324, calcd for C29H31O2 [M+H+] 411.2324; 433.2147, calcd for C29H30O2Na [M+Na+] 433.2144.
Synthesis of 4-tert-butyl-5-(1-hydroxyphenanthren-3-yl)-3,3-dimethyl-2,3-dihydrofuran (4-iv) : typical procedure. Sodium thiomethoxide (119 mg, 1.70 mmol) was added to a solution of 8-iv (301 mg, 0.835 mmol) in dry DMF (10 mL) and stirred under a nitrogen atmosphere at 150 °C for 30 min. The reaction mixture was poured into sat. aq. NH4Cl and extracted with AcOEt. The organic layer was washed with sat. aq. NaCl, dried over anhydrous MgSO4 and concentrated in vacuo. The residue was chromatographed on silica gel with AcOEt−hexane (5:1) to give 4-iv (286 mg, 0.854 mmol, 99%).
4-iv: colorless columns, mp 218.0−218.5 °C (from Et2O−hexane). 1H NMR (400 MHz, CDCl3): δΗ 1.10 (s, 9H), 1.41 (s, 6H), 3.97 (s, 2H), 5.40 (s, 1H), 6.92 (d , J = 1.2 Hz, 1H), 7.56−7.66 (m, 2H), 7.72 (d , J = 9.0 Hz, 1H), 7.88 (d with fine coupling, J = 7.6 Hz, 1H), 8.09 (dd, J = 9.0 and 0.6 Hz, 1H), 8.22 (s, 1H), 8.64 (d, J = 8.1 Hz, 1H) ppm. 13C NMR (125 MHz, CDCl3): δC 27.4, 32.6, 32.6, 47.3, 83.2, 112.6, 117.1, 119.9, 121.8, 123.2, 126.3, 126.4, 126.5, 126.6, 128.5, 130.0, 131.2, 132.2, 133.9, 149.8, 151.6 ppm. IR (KBr): ν̃ 3333, 2955, 2869, 1654, 1614, 1601 cm-1. MASS (m/z, %): 346 (M+, 29), 332 (24), 331 (100), 275 (17), 221 (20), 165 (14). HRMS (ESI): 369.1831, calcd for C24H26O2Na [M+Na+] 369.1831; 385.1574, calcd for C24H26O2K [M+K+] 385.1570.
4-ov: 97% yield. colorless columns, mp 139.0−139.5 °C (from CH2Cl2−hexane). 1H NMR (300 MHz, CDCl3): δH 1.01 (s, 9H), 1.43 (s, 3H), 1.50 (s, 3H), 4.02 (qAB, J = 8.1 Hz, 2H), 5.11 (s, 1H), 7.13 (d, J = 2.4 Hz, 1H), 7.54−7.61 (m, 2H), 7.62 (d, J = 8.9 Hz, 1H), 7.79 (d, J = 8.9 Hz, 1H), 7.82−7.87 (m, 1H), 8.00 (d, J = 2.4 Hz, 1H), 8.47−8.52 (m, 1H) ppm. 13C NMR (125 MHz, CDCl3): δC 27.3, 27.4, 31.9, 32.6, 47.3, 83.3, 107.8, 119.3, 122.8, 124.1, 124.9, 125.6, 126.1, 126.6, 128.3, 128.4, 129.5, 132.0, 132.2, 135.4, 147.2, 153.4 ppm. IR (KBr): ν̃ 3311, 2983, 2967, 2870, 1653, 1614, 1602 cm-1. Mass (m/z, %): 346 (M+, 3), 221 (10), 165 (49), 164 (13), 163 (15), 95 (25), 57 (100). HRMS (ESI): 369.1835, calcd for C24H26O2Na [M+Na+] 369.1831; 385.1579, calcd for C24H26O2K [M+K+] 385.1570.
4-oh: 98% yield. colorless columns, mp 179.5−180.0 °C (from AcOEt−hexane). 1H NMR (300 MHz, CDCl3): δH 1.10 (s, 9H), 1.39 (s, 6H), 3.94 (s, 2H), 5.77 (s, 1H), 6.95 (d, J = 1.5 Hz, 1H), 7.46 (d, J = 1.5 Hz, 1H), 7.54−7.68 (m, 2H), 7.66 (d, J = 8.9 Hz, 1H), 7.72 (d, J = 8.9 Hz, 1H), 7.87 (d with fine coupling, J = 7.6 Hz, 1H), 9.61 (d with fine coupling, J = 8.3 Hz, 1H) ppm. 13C NMR (125 MHz, CDCl3): δC 27.4, 32.5, 32.6, 47.3, 83.1, 114.9, 119.3, 122.9, 126.0, 126.4, 126.9 (broad), 127.0, 128.0, 128.1, 128.6, 130.1, 132.6, 133.7 (broad), 134.4, 149.1, 154.2 ppm. IR (KBr): ν̃ 3212, 3061, 2956, 2866, 1661, 1610, 1599, 1570 cm-1. Mass (m/z, %): 346 (M+, 31), 332 (24), 331 (100), 275 (16), 221 (16), 165 (11). HRMS (ESI): 347.2016, calcd for C24H27O2 [M+H+] 347.2011; 369.1829, calcd for C24H26O2Na [M+Na+] 369.1831. 385.1584, calcd for C24H26O2K [M+K+] 385.1570.
4-ih: 87% yield. colorless columns, mp 155.5−156.0 °C (from CH2Cl2−hexane). 1H NMR (500 MHz, CDCl3): δH 0.95 (s, 9H), 1.44 (s, 3H), 1.52 (s, 3H), 4.13 (qAB, J = 8.3 Hz, 2H) 5.87 (broad s, 1H), 6.91 (dd, J = 4.7 and 2.7 Hz, 1H), 7.07 (d, J = 2.8 Hz, 1H), 7.31 (dd, J = 8.8 and 4.7 Hz, 1H), 7.50 (dd with fine coupling, J = 7.7 and 6.9 Hz, 1H), 7.54 (dd with fine coupling, J = 8.4 and 6.9 Hz, 1H), 7.60 (d, J = 8.8 Hz, 1H), 7.80 (dd, J = 7.7 and 1.4 Hz, 1H), 9.13 (d, J = 8.4 Hz, 1H) ppm. 13C NMR (125 MHz, CDCl3): δC 26.1, 27.9, 31.6, 32.7, 47.1, 83.6, 113.4, 122.0, 123.3, 124.4, 125.5, 125.7, 126.3, 126.9, 127.9, 128.5, 130.4, 132.0, 133.9, 134.9, 150.6, 152.6 ppm. IR (KBr): ν̃ 3347, 3049, 2977, 2953, 2869, 1640, 1617, 1571 cm-1. Mass (m/z, %): 346 (M+, 27), 332 (24), 331 (100), 289 (13), 275 (11), 221 (12), 165 (11). HRMS (ESI): 369.1829, calcd for C24H26O2Na [M+Na+] 369.1831.
10-iv: 91% yield. colorless columns, mp 155.5−156.0 °C (from CH2Cl2−hexane). 1H NMR (300 MHz, CDCl3): δH 1.16 (s, 9H), 1.40 (s, 6H), 3.95 (s, 2H) 5.41 (broad s, 1H), 6.94 (d, J = 1.2 Hz, 1H), 7.58−7.70 (m, 2H), 7.81 (d, J = 8.4 Hz, 1H), 7.81 (d, J = 8.8 Hz, 1H), 7.90 (d, J = 8.4 Hz, 1H), 8.01 (d with fine coupling, J = 7.4 Hz, 1H), 8.28 (d with fine coupling, J = 8.8 Hz, 1H), 8.65 (d, J = 1.2 Hz, 1H), 9.08 (d with fine coupling, J = 8.0 Hz, 1H) ppm. 13C NMR (125 MHz, CDCl3): δC 27.4, 32.5, 32.6, 47.2, 83.0, 111.7, 120.6, 122.2, 123.8, 125.7, 125.9, 126.2, 126.6, 126.8, 127.2, 127.5, 128.1, 128.4, 130.4, 131.0, 131.3, 133.4, 133.4, 150.0, 151.6 ppm. IR (KBr): ν̃ 3396, 2957, 2921, 2868, 1651. 1606 cm-1. Mass (m/z, %): 396 (M+, 34), 382 (28), 381 (100), 325 (15), 271 (14), 215 (10). HRMS (ESI): 397.2178, calcd for C28H29O2 [M+H+] 397.2168; 419.1989, calcd for [M+Na+] 419.1987.
10-ov: 86% yield. colorless columns, mp 169.0−170.0 °C (from AcOEt−hexane). 1H NMR (500 MHz, CDCl3): δH 1.04 (s, 9H), 1.44 (s, 3H), 1.51 (s, 3H), 4.05 (qAB, J = 8.0 Hz, 2H), 5.39 (s, 1H), 7.17 (d, J = 2.5 Hz, 1H), 7.57 (dd with fine coupling, J = 7.8 and 6.9 Hz, 1H), 7.61 (dd with fine coupling, J = 8.3 and 6.9 Hz, 1H), 7.68 (d, J = 8.8 Hz, 1H), 7.76 (d, J = 8.5 Hz, 1H), 7.84 (d, J = 8.5 Hz, 1H), 7.95 (d, J = 8.8 Hz, 1H), 7.97 (d with fine coupling, J = 7.8 Hz, 1H), 8.46 (d, J = 2.5 Hz, 1H), 9.03 (d, J = 8.3 Hz, 1H) ppm. 13C NMR (125 MHz, CDCl3): δC 27.3, 27.4, 31.9, 32.7, 47.3, 83.3, 112.6, 118.8, 124.7, 124.9, 125.5, 125.9, 126.3, 126.7, 127.3, 127.3, 127.5, 128.4, 128.4, 130.4, 131.3, 131.8, 133.2, 135.2, 147.4, 153.2 ppm. IR (KBr): ν̃ 3348, 3051, 2958, 2869, 1650, 1603 cm-1. Mass (m/z, %): 396 (M+, 2), 324 (10), 295 (4), 271 (12), 226 (11), 215 (33), 213 (37), 57 (100). HRMS (ESI): 419.1992, calcd for C28H28O2Na [M+Na+] 419.1987; 435.1741, calcd for C28H28O2K [M+K+] 435.1726.
Synthesis of 5-tert-butyl-1-(1-hydroxyphenanthren-3-yl)-4,4-dimethyl-2,6,7-trioxabicyclo[3.2.0]heptane (2-iv) : typical procedure. A solution of 4-iv (201 mg, 0.580 mmol) and tetraphenylporphin (TPP) (2.0 mg) in CH2Cl2 (20 mL) was irradiated externally with a 940 W Na lamp under an O2 atmosphere at 0 °C for 35 min. The residue was chromatographed on silica gel with Et2O−hexane (1:9) to give 2-iv (171 mg, 0.452 mmol, 78%) to give colorless solid.
2-iv: colorless columns, mp 99.5−100.5 °C (from Et2O−hexane). 1H NMR (400 MHz, CDCl3): δΗ 1.02 (s, 9H), 1.21 (s, 3H), 1.47 (s, 3H), 3.91 (d, J = 8.2 Hz, 1H), 4.68 (d, J = 8.2 Hz, 1H), 5.53 (s, 1H), 7.21 (s, 1H), 7.62 (dd with fine coupling, J = 7.8 and 7.0 Hz, 1H), 7.67 (dd with fine coupling, J = 8.2 and 7.0 Hz, 1H), 7.80 (d , J = 9.1 Hz, 1H), 7.91 (dd, J = 7.8 and 1.1 Hz, 1H), 8.13 (d, J = 9.1 Hz, 1H), 8.61 (s, 1H), 8.71 (d, J = 8.2 Hz, 1H) ppm. 13C NMR (125 MHz, CDCl3): δC 18.5, 25.2, 26.9, 36.9, 45.7, 80.3, 105.2, 110.1, 116.1, 116.9, 119.7, 122.5, 123.3, 126.9, 127.0, 127.4, 128.7, 130.2, 131.1, 132.3, 134.1, 151.7 ppm. IR (KBr): ν̃ 3419, 3238, 2979, 2895, 1620, 1603, 1576 cm-1. Mass (m/z, %): 378 (M+, 20), 322 (18), 238 (36), 221 (100) 193 (16), 165 (26), 57 (41). HRMS (ESI): 401.1734, calcd for C24H26O4Na [M+Na+] 401.1729.
2-ov: 96% yield. colorless columns, mp 163.0−163.5 °C (from CH2Cl2−hexane). 1H NMR (500 MHz, CDCl3): δH 0.92 (s, 9H), 1.32 (s, 3H), 1.67 (s, 3H), 4.12 (d, J = 8.7 Hz, 1H), 4.72 (d, J = 8.7 Hz, 1H), 5.17 (broad s 1H), 7.57−7.65 (m, 2H), 7.63 (d, J = 9.4 Hz, 1H), 7.83−7.87 (m, 1H), 7.97 (broad, 1H), 8,21 (d, J = 2.8 Hz, 1H), 8.38−8.48 (m, 1H), 8.57 (d, J = 7.8 Hz, 1H) ppm. 13C NMR (125 MHz, CDCl3): δC 20.2, 26.2, 26.8, 36.9, 45.7, 80.8, 106.5, 109.3, 117.3, 120.2, 123.1, 124.3, 124.4, 124.9, 126.4, 127.0, 128.2, 129.8, 131.6, 133.2, 133.9, 153.1 ppm. IR(KBr): ν̃ 3452, 2975, 2891, 1614, 1405, 1217 cm-1. Mass (m/z, %): 378 (M+, 39), 322 (20), 238 (50), 222 (16), 221 (100). HRMS (ESI): 401.1726, calcd for C24H26O4Na [M+Na+] 401.1729; 417.1504, calcd for C24H26O4K [M+K+] 417.1468.
2-oh: 68% yield. pale yellow columns, mp 140.5−141.5 °C (from CH2Cl2−hexane). 1H NMR (400 MHz, CDCl3): δH 1.02 (s, 9H), 1.20 (s, 3H), 1.46 (s, 3H), 3.90 (d, J = 8.3Hz, 1H), 4.66 (d, J = 8.3 Hz, 1H), 5.83 (s, 1H), 7.25 (d, J = 1.6 Hz, 1H), 7.59−7.70 (m, 2H), 7.73 (d, J = 8.9 Hz, 1H), 7.77 (d, J = 8.9 Hz, 1H), 7.80 (d, J = 1.6 Hz, 1H), 7.90 (d with fine coupling, J = 7.8 Hz, 1H), 9.64 (d with fine coupling, J = 8.4 Hz, 1H) ppm. 13C NMR (125 MHz, CDCl3): δC 18.5, 25.1, 26.9, 36.8, 45.7, 80.3, 105.3, 112.5, 116.6, 120.1, 121.8, 126.4, 126.7, 127.3, 128.2, 128.5, 128.7, 129.9, 133.0, 133.8, 134.3, 154.3 ppm. IR (KBr): ν̃ 3245, 3051, 2968, 1627, 1574, 1402 cm-1. Mass (m/z, %): 378 (M+, 24), 322 (19), 238 (39), 222 (17), 221 (100), 193 (19), 165 (51). HRMS (ESI): 401.1719, calcd for C24H26O4Na [M+Na+] 401.1729.
3-iv: 60% yield. pale yellow columns mp 190.0−91.0 °C (from CH2Cl2−hexane). 1H NMR (300 MHz, CDCl3): δH 1.07 (s, 9H), 1.21 (s, 3H), 1.50 (s, 3H), 3.89 (d, J = 8.2 Hz, 1H), 4.67 (d with fine coupling, J = 8.2 Hz, 1H), 5.49 (s 1H), 7.25 (broad d, J = 1.1 Hz, 1H), 7.59−7.70 (m, 2H), 7.83 (d, J = 8.5 Hz, 1H), 7.89 (d, J = 8.8 Hz, 1H), 7.92 (d, J = 8.5 Hz, 1H), 7.99−8.05 (m, 1H), 8.33 (d with fine coupling, J = 8.8 Hz, 1H), 9.01−9.07 (m, 2H) ppm. 13C NMR (125 MHz, CDCl3): δC 18.5, 25.0, 27.0, 36.9, 45.7, 80.3, 105.5, 109.1, 117.2, 120.5, 121.0, 124.3, 126.0, 126.2, 126.7, 127.2, 127.5, 127.9 (x 2), 128.5, 130.3, 130.8, 131.5, 133.5, 133.6, 151.8 ppm. IR (KBr): ν̃ 3439, 3052, 3005, 2964, 2898, 1613, 1603, 1413 cm-1. Mass (m/z, %): 428 (M+, 62), 372 (31), 288 (39), 272 (22), 271 (100), 57 (43). HRMS (ESI): 451.1895, calcd for C28H28O4Na [M+Na+] 451.1885.
3-ov: 86% yield. pale yellow columns, mp 192.0−193.0 °C (from AcOEt−hexane). 1H NMR (400 MHz, CDCl3): δH 0.95 (s, 9H), 1.33 (s, 3H), 1.69 (s, 3H), 4.14 (d, J = 8.5 Hz, 1H), 4.74 (d, J = 8.5 Hz, 1H), 5.21 (s 1H), 7.58−7.70 (m, 2H), 7.70 (d, J = 9.0 Hz, 1H), 7.77 (d, J = 8.5 Hz, 1H), 7.89 (d, J = 8.5 Hz, 1H), 7.96−8.03 (m, 1H), 8.00 (dd, J = 7.9 and 1.3 Hz, 1H), 8.56−8.64 (m, 1H), 8.62 (d, J = 2.2 Hz, 1H), 9.04 (d, J = 8.3 Hz, 1H) ppm. 13C NMR (125 MHz, CDCl3): δC 20.2, 26.2, 26.9, 37.0, 45.7, 80.8, 106.4, 114.3, 119.9, 124.4, 124.8, 125.8, 126.2, 126.4, 126.6, 126.8, 127.7, 128.0, 128.6, 127.7, 128.0, 128.6, 130.4, 130.7, 132.9, 133.4, 133.6, 152.6 ppm. IR (KBr): ν̃ 3460, 3050, 2998, 2977, 2896, 1616 cm-1. Mass (m/z, %): 428 (M+, 63), 372 (24), 288 (58) 272 (22), 271 (100), 215 (30), 57 (30). HRMS (ESI): 429.2110, calcd for C28H29O4 [M+H+] 429.2066; 451.1876, calcd for C28H28O4Na [M+Na+] 451.1885.
Synthesis of 2,2,4,4-tetramethyl-3-oxopentyl 1-hydroxyphenanthrene-3-carboxylate (9-iv): typical procedure. TBAF (1M in THF, 0.75 mL, 0.75 mmol) in MeCN (1.5 mL) was added to a solution of 2-iv (57.0 mg, 0.151 mmol) in MeCN (1.5 mL) and stirred for 5 min. The reaction mixture was poured into sat. aq. NH4Cl and extracted with AcOEt. The organic layer was washed with sat. aq. NaCl, dried over anhydrous MgSO4 and concentrated in vacuo. The residue was chromatographed on silica gel and recrystallization with Et2O−hexane (1:3) gave 9-iv (53.0 mg, 0.140 mmol, 93%).
9-iv: pale yellow needles, mp 164.5−165.0 °C (from CH2Cl−hexane). 1H NMR (400 MHz, CDCl3): δΗ 1.35 (s, 9H), 1.47 (s, 6H), 4.53 (s, 2H), 6.13 (s, 1H), 7.58 (s with fine coupling, 1H), 7.64 (dd with fine coupling, J = 7.3 and 7.1 Hz, 1H), 7.69 (dd with fine coupling, J = 8.2 and 7.1 Hz, 1H), 7.86 (d , J = 9.1 Hz, 1H), 7.92 (d, J = 7.3 Hz, 1H), 8.18 (d, J = 9.1 Hz, 1H), 8.67 (d, J = 8.2 Hz, 1H), 8.96 (s, 1H) ppm. 13C NMR (125 MHz, CDCl3): δC 23.8, 28.2, 46.0, 49.5, 72.4, 110.0, 117.0, 120.1, 123.0, 125.3, 127.0, 127.1, 127.4, 128.5, 128.7, 130.3, 131.1, 132.2, 152.8, 167.0, 217.1 ppm. IR (KBr): ν̃ 3433, 2971, 1695, 1604, 1274 cm-1. Mass (m/z, %): 378 (M+, 31), 322 (24), 238 (36), 222 (16), 221 (100), 193 (13), 165 (20), 57 (31). HRMS (ESI): 401.1741, calcd for C24H26O4Na [M+Na+] 401.1729.
9-ov: colorless columns, mp 163.0−163.5 °C (from CH2Cl2−hexane). 1H NMR (400 MHz, CDCl3): δΗ 1.29 (s, 9H), 1.44 (s, 6H), 4.51 (s, 2H), 5.90 (s, 1H), 7.58−7.66 (m, 2H), 7.69 (d, J = 2.6 Hz, 1H), 7.69 (d, J = 9.4 Hz, 1H), 7.84−7.90 (m, 1H), 8.28 (d, J = 2.6 Hz, 1H), 8.52−8.57 (m, 1H), 8.63 (d, J = 9.4 Hz, 1H) ppm. 13C NMR (125 MHz, CDCl3): δC 23.7, 28.2, 46.0, 49.3, 72.5, 111.7, 119.5, 122.8, 123.5, 125.3, 126.3, 126.4, 127.1, 128.4, 129.2, 129.5, 131.9, 132.8, 153.1, 167.2, 216.9 ppm. IR (KBr): ν̃ 3420, 2965, 2928, 1714, 1674, 1620, 1229 cm-1. Mass (m/z, %): 378 (M+, 40), 322 (21), 238 (50), 222 (16), 221 (100), 165 (33), 57 (22). HRMS (ESI): 401.1728, calcd for C24H26O4Na [M+Na+] 401.1729.
9-oh: yellow columns, mp 163.0−163.5 °C (from CH2Cl2−hexane). 1H NMR (400 MHz, CDCl3): δH 1.33 (s, 9H), 1.45 (s, 6H), 4.51 (s, 2H), 6.35 (broad s, 1H), 7.60 (d, J = 1.6 Hz, 1H), 7.62 (dd with fine coupling, J = 7.8 and 7.0 Hz, 1H), 7.67 (dd with fine coupling, J = 8.3 and 7.0 Hz, 1H), 7.73 (d, J = 8.9 Hz, 1H), 7.78 (d, J = 8.9 Hz, 1H), 7.91 (dd, J = 7.4 and 1.6 Hz, 1H), 8.13 (d, J = 1.6 Hz, 1H), 9.70 (d, J = 8.4 Hz, 1H) ppm. 13C NMR (125 MHz, CDCl3): δC 23.7, 28.2, 46.0, 49.4, 72.4, 112.7, 122.9, 123.0, 126.7, 126.9, 127.1, 127.4, 128.2, 128.6, 129.1, 130.0, 133.4, 134.2, 155.5, 166.9, 217.2 ppm. IR (KBr): ν̃ 3356, 2976, 1720, 1690, 1669, 1227 cm-1. Mass (m/z, %): 378 (M+,1), 238 (2), 221 (4), 165 (18), 163 (11), 57 (100). HRMS (ESI): 401.1728, calcd for C24H26O4Na [M+Na+] 401.1729.
9-ih: colorless columns, mp 148.5−149.0 °C (from CH2Cl2−hexane). 1H NMR (400 MHz, CDCl3): δH 1.07 (s, 9H), 1.29 (s, 6H), 4.99 (s, 2H), 5.48 (broad s, 1H), 7.25 (d, J = 2.7 Hz, 1H), 7.30 (d, J = 8.8 Hz, 1H), 7.81−7.85 (m, 1H), 8.07−8.11 (m, 1H) ppm. 13C NMR (125 MHz, CDCl3): δC 23.6, 27.7, 45.8, 48.9, 73.7, 114.6, 117.8, 121.6, 125.5, 125.9, 126.0, 126.4, 128.4, 128.5, 128.9, 131.9, 134.8, 153.3, 171.9, 216.7 ppm. IR (KBr) : ν̃ 3371, 2981, 2875, 1720, 1667, 1621 cm-1. Mass (m/z, %): 378 (M+, 1), 221 (4) 193 (6), 165 (19), 57 (100). HRMS (ESI): 401.1733, calcd for C24H26O4Na [M+Na+] 401.1729.
14-iv: yellow needles, mp197.0−198.0 °C (from AcOEt−hexane). 1H NMR (400 MHz, CDCl3): δH 1.27 (s, 9H), 1.46 (s, 6H), 4.53 (s, 2H), 5.95 (broad s, 1H), 7.60 (d, J = 1.3 Hz, 1H), 7.66 (dd with fine coupling, J = 7.9 and 7.0 Hz, 1H), 7.76 (dd with fine coupling, J = 8.5 and 7.0 Hz, 1H), 7.84 (d, J = 8.5 Hz, 1H), 7.94 (d, J = 8.5 Hz, 1H) 7.95 (d, J = 8.8 Hz, 1H), 8.03 (dd, J = 7.9 and 1.4 Hz, 1H), 8.37 (d with fine coupling, J = 8.8 Hz, 1H), 9.05 (d, J = 8.5 Hz, 1H), 9.44 (s with fine coupling, 1H) ppm. 13C NMR (125 MHz, CDCl3): δC 23.6, 28.3, 45.9, 49.4, 72.1, 108.8, 120.8, 122.9, 126.1, 126.6, 126.6, 126.8, 127.0, 127.8, 128.0, 128.1, 128.5, 128.5, 130.1, 130.8, 131.4, 133.6, 152.7, 167.3, 216.8 ppm. IR (KBr): ν̃ 3416, 3048, 2976, 2964, 1698, 1603, 1418, 1278 cm-1. Mass (m/z, %): 428 (M+, 58), 372 (30), 288 (43), 272 (20), 271 (100), 243 (16), 242 (11), 215 (18), 213 (15), 57 (28). HRMS (ESI): 451.1888, calcd for C28H28O4Na [M+Na+] 451.1885.
14-ov: colorless columns, mp 133.0−133.5 °C (from CH2Cl2−hexane). 1H NMR (500 MHz, CDCl3): δH 1.30 (s, 9H), 1.45 (s, 6H), 4.53 (s, 2H), 5.84 (s, 1H), 7.60 (ddd, J = 7.8, 6.9 and 1.1 Hz, 1H), 7.65 (ddd, J = 8.2, 6.9 and 1.6 Hz, 1H), 7.75 (d, J = 8.9 Hz, 1H), 7.77 (d, J = 2.5 Hz, 1H), 7.78 (d, J = 8.5 Hz, 1H), 7.90 (d, J = 8.5 Hz, 1H), 8.00 (dd, J = 7.8 and 1.6 Hz, 1H), 8.70 (d, J = 2.5 Hz, 1H), 8.75 (d, J = 8.9 Hz, 1H), 8.96 (d, J = 8.2 Hz, 1H) ppm. 13C NMR (125 MHz, CDCl3): δC 23.7, 28.2, 46.0, 49.3, 72.5, 116.6, 119.3, 124.3, 125.8, 126.2, 126.3, 126.3, 126.5, 126.9, 127.4, 128.1, 128.6, 129.2, 130.2, 131.1, 132.5, 133.4, 152.7, 167.2, 216.6 ppm. IR (KBr):ν̃ 3341, 2979, 2959, 1687, 1603, 1210 cm-1. Mass (m/z, %): 428 (M+, 61), 372 (23), 289 (12), 288 (57), 272 (22), 271 (100), 243 (11), 242 (11), 215 (30), 213 (17), 57 (25). HRMS (ESI): 451.1878, calcd for C28H28O4Na [M+Na+] 451.1885.
Chemiluminescence measurement and time-course for the charge-transfer-induced decomposition of dioxetane. general procedure: Chemiluminescence was measured using a spectrophotometer and/or a multi-channel detector.
A freshly prepared solution (2.0 mL) of TBAF (1.0 x 10-2 mol dm-3) in MeCN was transferred to a quartz cell (10 x 10 x 50 mm) and the latter was placed in a spectrometer, which was thermostated with stirring at 25 °C. After ca. 1 min, a solution of dioxetane 2 or 3 in MeCN (1.0 x 10-4 or 1.0 x 10-5 mol dm-3, 1.0 mL) was added by a syringe, and measurement was started immediately. The time-course of the intensity of light emission was recorded and processed according to first-order kinetics. The total light emission was estimated by comparing it to that of an adamantylidene-dioxetane, the chemiluminescence efficiency ΦCL of which has been reported to be 0.29 and which was used here as a standard.15,16
Fluorescence Measurement of Authentic Emitters 9 and 14: A freshly prepared solution of 2.0 x 10-5 ~ 3.0 x 10-4 mol dm-3 of 9 or 14 and 1.0 x 10-3 mol dm-3 of TBAF in MeCN was transferred to a quartz cell (10 x 10 x 50 mm), and the latter was placed in the spectrometer, which was thermostated with stirring at 25 °C. Thus, the fluorescence spectra of 9 and 14 were measured and their fluorescence efficiencies (Φfl) were estimated using quinine hydrosulfate as a standard.
X-Ray single crystallographic analysis of 3-iv and 3-ov
X-Ray diffraction data were collected on a Rigaku Mercury CCD diffractometer with graphite monochromated Mo Kα (λ=0.71070 Å) radiation. Data were processed using CrystalClear.† The structures were solved by direct method (SIR2008)‡ and expanded using Fourier techniques. The non-hydrogen atoms were refined anisotropically. Hydrogen atoms were refined using the riding model. The final cycle of full-matrix least-squares refinement on F2 was based on 5094 observed reflections and 318 variable parameters (for 3-iv) and on 5009 observed reflections and 318 variable parameters (for 3-ov). All calculations were performed using the CrystalStructure crystallographic software package.§,¶
† Rigaku Corporation, 1999.
‡ SIR2008: M.C. Burla, R. Caliandro, M. Camalli, B. Carrozzini, G.L. Cascarano, L.
De Caro, C. Giacovazzo, G. Polidori, D. Siliqi, R. Spagna (2007).
§ CrystalStructure 4.0: Crystal Structure Analysis Package, Rigaku Corporation
(2000-2010). Tokyo 196-8666, Japan.
¶ CRYSTALS Issue 11: Carruthers, J.R., Rollett,J.S., Betteridge, P.W., Kinna, D.,
Pearce, L., Larsen, A., and Gabe, E. Chemical Crystallography Laboratory,
Oxford, UK. (1999).
Crystal data for 3-iv: C28H28O4 (Mr = 428.53), pale yellow block, 0.12 x 0.10 x 0.05 mm, monoclinic, space group P21/c (#14), a = 16.45(5) Å, b = 12.31(2) Å, c = 11.029(17) Å, β = 90.101(19)°, V = 2233(9) Å3, Z = 4, ρcalcd = 1.274 g cm-3, T = 120 K, F(000) = 912.00, reflections collected/unique 23562 / 5094 (Rint = 0.0587), μ(MoKα) = 0.84 cm-1. An empirical absorption correction was applied which resulted in transmission factors ranging from 0.898 – 1.0000. The data were corrected for Lorentz and polarization effects. R1 = 0.062 [ I >2σ(I)], wR2= 0.186 (all data) GOF on F2 = 1.004, and residual electron density 0.43 / -0.43 e-Å-3.
Crystal data for 3-ov: C28H28O4 (Mr = 428.53), pale yellow prism, 0.15 x 0.10 x 0.07 mm, monoclinic, space group P21/c (#14), a = 16.75(3) Å, b = 12.336(11) Å, c = 11.202(12) Å, β = 108.40(13)°, V = 2196(5) Å3, Z = 4, ρcalcd = 1.296 g cm-3, T = 120 K, F(000) = 912.00, reflections collected/unique 22506 / 5009 (Rint = 0.051), μ(MoKα) = 0.854 cm-1. An empirical absorption correction was applied which resulted in transmission factors ranging from 0.923 – 1.0000. The data were corrected for Lorentz and polarization effects. R1 = 0.0454 [ I >2σ(I)], wR2= 0.0984 (all data) GOF on F2 = 1.002, and residual electron density 0.34 / -0.33 e-Å -3.
Crystallographic data for the structural analysis of compound 3-iv and 3-ov have been deposited at the Cambridge Crystallographic Data Center, CCDC-989777 and CCDC-989778. Copies of the data can be obtained, free of charge, on application to CCDC, 12 Union Road, Cambridge CB2 1EZ, UK, (fax: +44-(0)1223-336033 or e-mail: deposit@ccdc.cam.ac.uk).
ACKNOWLEDGEMENTS
We gratefully acknowledge financial assistance provided by Grants-in-aid (No. 25350269 No. 25410056 and No. 25410057) for Scientific Research from the Ministry of Education, Culture, Sports, Science, and Technology, Japan.
References
1. A. P. Schaap and S. D. Gagnon, J. Am. Chem. Soc., 1982, 104, 3504. CrossRef
2. (a) A. P. Schaap, R. S. Handley, and B. P. Giri, Tetrahedron Lett., 1987, 28, 935; CrossRef (b) A. P. Schaap, T. S. Chen, R. S. Handley, R. DeSilva, and B. P. Giri, Tetrahedron Lett., 1987, 28, 1155. CrossRef
3. (a) S. Beck and H. Köster, Anal. Chem., 1990, 62, 2258; CrossRef (b) W. Adam, D. Reihardt, and C. R. Saha-Möller, Analyst., 1996, 121, 1527; CrossRef (c) M. Matsumoto, J. Photochemphotobiol. C: Photochem. Rev., 2004, 5, 27; (d) M. Matsumoto and N. Watanabe, Bull. Chem. Soc. Jpn., 2005, 78, 1899. CrossRef
4. (a) W. Adam and A. V. Trofimov, In The Chemistry of Peroxides; ed. by Z. Rappoport; Wiley: New York, 2006; Vol. 2, pp. 1171–1209; CrossRef (b) W. J. Baader, C. V. Stevani, and E. L. Bastos, In The Chemistry of Peroxides; ed. by Z. Rappoport; Wiley: New York, 2006; Vol. 2, pp. 1211–1278. CrossRef
5. (a) A. V. Trofimov, R. F. Vasil’ev, K. Mielke, and W. Adam, Photochem Photobiol., 1995, 62, 35; CrossRef (b) W. Adam, I. Bronstein, B. Edwards, T. Engel, D. Reinhardt, F. W. Schneider, A. V. Trofimov, and R. F. Vasil’ev, J. Am. Chem. Soc., 1996, 118, 10400; CrossRef (c) W. Adam, I. Bronstein, A. V. Trofimov, and R. F. Vasil’ev, J. Am. Chem. Soc., 1999, 121, 958.
6. (a) M. Matsumoto, N. Watanabe, N. C. Kasuga, F. Hamada, and K. Tadokoro, Tetrahedron Lett., 1997, 38, 2863; CrossRef (b) W. Adam, M. Matsumoto, and A. V. Trofimov, J. Am. Chem. Soc., 2000, 122, 8631. CrossRef
7. (a) I. Bronstein, B. Edwards, and J. C. Voyta, J. Biolumin. Chemilumin., 1989, 4, 99; CrossRef (b) A. P. Schaap, H. Akhavan, and R. J. Romano, Clin. Chem., 1989, 35, 1863.
8. M. Yamada, K. Kitaoka, M. Matsumoto, and N. Watanabe, In Bioluminescence and Chemiluminescence, ed. by A. Tsuji, M. Matsumoto, M. Maeda, L. J. Kricka, and P. E. Stanley; World Scientific: Singapore, 2004; pp. 487–490.
9. (a) M. Matsumoto, K. Hamaoka, Y. Takashima, M. Yokokawa, K. Yamada, N. Watanabe, and H. K. Ijuin, Chem. Commun., 2005, 808; CrossRef (b) N. Hoshiya, N. Watanabe, H. K. Ijuin, and M. Matsumoto, Tetrahedron, 2006, 62, 12424. CrossRef
10. M. Matsumoto, N. Watanabe, N. Hoshiya, and H. K. Ijuin, Chem. Rec., 2008, 8, 213. CrossRef
11. (a) B. Edward, A. Sparks, J. C. Voyta, and I. Bronstein, J. Biolumin. Chemilumin., 1990, 5, 1; CrossRef (b) B. Edward, A. Sparks, J. C. Voyta, Strong, O. Murphy, and I. Bronstein, J. Org. Chem., 1990, 55, 6225; (c) N. Watanabe, H. Kobayashi, M. Azami, and M. Matsumoto, Tetrahedron, 1999, 55, 6831; CrossRef (d) N. Hoshiya, N. Hukuda, H. Maeda, N. Watanabe, and M. Matsumoto, Tetrahedron, 2006, 62, 5808. CrossRef
12. An “odd/even” relationship between the chemiluminescence properties and substitution pattern of a trigger group for CTID of oxyaryl-substituted dioxetanes was initially observed for dioxetanes bearing a 6- or 7-oxynaphthyl group. An “odd” pattern is one which the donor’s (-O−) point of attachment to the acceptor (dioxetane ring) is such that the total number of ring carbon atoms separating these points, including the atoms at the point of attachment, is an odd whole number [Refs. 11(a) and 11(b)].
13. For convenience, we refer to the four hydroxyphenanthryl-substituted dioxetanes investigated here as follows. For the structural formula shown in Figure 2, there are two types of isomers, i.e., one 2-v possesses a phenanthrene C ring that lies vertically and the other 2-h has a ring that lies horizontally with respect to the dioxetane ring. Second, these two isomers can each be separated into two isomers in which the helix of the phenanthrene ring is oriented inner or outer with respect to the dioxetane ring. Thus, there are four possible types, i.e., 2-iv, 2-ov, 2-oh, and 2-ih, for dioxetane 2. A similar discussion can be applied to dioxetanes 3-iv, and 3-ov.
14. C. D. Harrowven, L. L. Guy, and L. Nanson, Angew. Chem. Int. Ed., 2006, 45, 2242. CrossRef
15. All of the ΦCL values presented here were estimated based on the value reported for the chemiluminescent decomposition of 3-adamantylidene-4-(3-siloxyphenyl)-4-methoxy-1,2-dioxetane (ΦCL = 0.29) in TBAF/DMSO16.
16. A. V. Trofimov, K. Mielke, R. F. Vasil'ev, and W. Adam, Photochem. Photobiol., 1996, 63, 463. CrossRef
17. M. Matsumoto, Y. Mizoguchi, T. Motoyama, and N. Watanabe, Tetrahedron Lett., 2001, 42, 88692.
18. A. Nishinaga, M. Yano, T. Kuwashige, K. Maruyama, and T. Mashino, Chem. Lett., 1994, 817. CrossRef
19. For convenience sake, we call a rotamer an anti-form when the dioxetane O-O and a hydroxy group on an aromatic ring are on opposite sides of the furan ring, while in the syn-form the dioxetane O-O and a hydroxy group on an aromatic ring are on the same side of the furan ring.
20. (a) M. Matsumoto, H. Suzuki, N. Watanabe, H. K. Ijuin, J. Tanaka, and C. Tanaka, J. Org. Chem., 2011, 76, 5006; CrossRef (b) N. Watanabe, K. Matsumoto, T. Tanaka, H. Suzuki, H. K. Ijuin, and M. Matsumoto, Tetrahedron Lett., 2012, 53, 5309. CrossRef