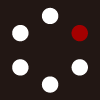
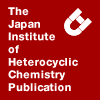
HETEROCYCLES
An International Journal for Reviews and Communications in Heterocyclic ChemistryWeb Edition ISSN: 1881-0942
Published online by The Japan Institute of Heterocyclic Chemistry
e-Journal
Full Text HTML
Received, 27th February, 2014, Accepted, 25th March, 2014, Published online, 3rd April, 2014.
DOI: 10.3987/REV-14-SR(K)1
■ Prenylated Acylphloroglucinols and Meroterpenoids from Hypericum Plants
Naonobu Tanaka and Jun'ichi Kobayashi*
Graduate School of Pharmaceutical Sciences, Hokkaido University, Kita 12 Nishi 6, Kita-ku, Sapporo, Hokkaido 060-0812, Japan
Abstract
Hypericum plants {family Clusiaceae (APG: Hypericaceae)} are known to be a rich source of prenylated acylphloroglucinols (PAPs), some of which have attracted attention as new drug leads as well as challenging targets for total synthesis. This review covers the researches of the isolation and structure elucidation of PAPs from Hypericum plants from 2006 to 2013. Several meroterpenoids with interesting chemical structures isolated from this genus are also summarized.INTRODUCTION
The plants of the genus Hypericum {family Clusiaceae (APG: Hypericaceae)} are divided into 50 sections including more than 400 species, which are widespred in temperate regions. Several of the botanical species belonging to the genus have been used in traditional remedies.1 Hypericum perforatum (St. John’s Wort) counts among the most favorite herbal drugs, and is the only herbal alternative to classic synthetic antidepressants in the therapy of mild to moderate depression.2 Pharmacological activities of Hypericum plants inspired many researchers to investigate the constituents, resulting in the isolation of a variety of natural products such as essential oils, flavonoids, xanthones, naphthodianthrones, terpenoids, and prenylated acylphloroglucinols (PAPs).1 PAPs are characteristic constituents of the Clusiaceae plant family, and several compounds of this class (e.g. hyperforin from H. perforatum) have fascinating chemical structures and intriguing bioactivities, and are paid attention as a new drug lead from plant sources. The biosysnthesis of PAPs is proposed as follows.3 Condensation of three molecules of malonyl-CoA and one molecule of acyl-CoA gives a tetraketide, which is subsequently cyclized by Dieckmann condensation into an acylphloroglucinol. The alkylations such as prenylation, geranylation, and methylation of the acylphloroglucinol afford PAPs. In 2006, reviews for the occurrence, chemical structure, and biological activity of natural PAPs were summarized by Grossman et al.3 and Singh et al.4
This review covers the reports on PAPs isolated from Hypericum plants that have been published from 2006 to 2013. During this period, 115 PAPs from 21 species (H. androsaemum, H. ascyron, H. beanii, H. carinatum, H. chinense, H. cohaerens, H. elegans, H. ellipticum, H. empetrifolium, H. erectum, H. foliosum, H. geminiflorum, H. henryi subsp. uraloides, H. hypericoides, H. olympicum, H. prolificum, H. pseudopetiolatum var. kiusianum, H. sampsonii, H. sikokumontanum, H. sinaicum, and H. yojiroanum) were reported. In this review, PAPs are classified into sections (A) simple PAPs with mono- to tetra-cyclic structures, (B) O-prenylated acylphloroglucinols, (C) PAPs with spiro-structures, (D) polycyclic polyprenylated acylphloroglucinols (PPAPs), and (E) PPAPs with caged-structures. In addition, meroterpenoids isolated from Hypericum plants are also summarized in the section (F).
A. SIMPLE PRENYLATED ACYLPHLOROGLUCINOLS
Elliptophenone A (1), a simple prenylated benzoylphloroglucinol with two prenyl groups and one methyl group, was isolated from the aerial portions of Hypericum ellipticum5 (Figure 1). This compound showed a weak cytotoxicity against three human colon cancer cell lines (HT-29, HCT-116, and Caco-2) as well as a normal colon cell line (CCD-18Co). An acylphloroglucinol aspidinol C (2) (leaves of H. chinense)6 exhibited antimicrobial activity against the NorA efflux protein overexpressing MDR Staphylococcus aureus strain SA-1199B with a minimum inhibitory concentration (MIC) of 2 µg/mL. A PAP (3) (aerial parts of H. foliosum)7 demonstrated antibacterial activity against a panel of multidrug-resistant strains of S. aureus (MRSA) with MICs ranged from 16 to 32 µg/mL. Empetrikathiforin (4) was obtained from the aerial parts of H. empetrifolium.8
Bicyclic PAPs possessing chromane skeleton such as cariphenones A (5) and B (6) (leaves of H. carinatum),9 empetrikarinens A (7) and B (8) and empetrikarinols A (9) and B (10) (aerial parts of H. empetrifolium),10 petiolins A (11), B (12), and J (13) (aerial parts of H. pseudopetiolatum var. kiusianum),11,12 yojironins D (14) and I (15) (whole plants of H. yojiroanum),13,14 otogirinins F (16) and G (17) (aerial parts of H. erectum),15 and prolificin A (18) (aerial parts of H. prolificum)16 were reported (Figure 2). Cariphenones A (5) and B (6) have a benzoyl group as the acyl moiety, and 5 showed a moderate antioxidant activity. Empetrikarinens A (7) and B (8) and petiolins A (11) and B (12) are geranylated acylphloroglucinols, and 11 and 12 possess a geminal dimethyl group at the phloroglucinol moiety. Petiolin J (13) exhibited antimicrobial activities against Micrococcus luteus, Cryptococcus neoformans, and Trichophyton mentagrophytes. Otogirinin F (16) displayed a weak suppressive activity against isovaleric acid generation from L-leucine by Corynebacterium xerosis. Prolificin A (18) showed a moderate growth inhibition of human tumor cell lines (MCF-7, NCI-H460, SF-268, AGS, and HCT-116).
The structures of bicyclic PAPs having a dihydrofuran ring, petiolins C (19), L (20), and M (22) (aerial parts of H. pseudopetiolatum var. kiusianum),11,12 yojironins C (21), E (23), F (24), G (25), and H (26) (whole plants of H. yojiroanum),13,14 and takaneols A (27) and B (28) (aerial parts of H. sikokumontanum),17 are shown in Figure 3. Their structures were assigned on the basis of spectroscopic analysis. Petiolin C (19) and yojironin E (23) showed antimicrobial activities, while takaneols A (27) and B (28) exhibited a moderate cytotoxicity against human cancer cell lines.
Empetriferdinans A (29) and B (30) and empetriferdinol (31) are tricyclic PAPs found in the aerial parts of H. empetrifolium10 (Figure 4). The ECD spectra of 29–31 suggested that the absolute stereochemistry of their tricyclic ring system was identical. Tetracyclic PAPs with citran skeleton, petiolins D (32) and K (33) (aerial parts of H. pseudopetiolatum var. kiusianum)18,12 and empetrifranzinans A–C (34–36) (aerial parts of H. empetrifolium)10 were isolated. X-Ray analysis and optical rotations of 32 and 33 suggested that they were racemates, whereas 36 was optically active. Empetrifranzinans A (34) and B (35) were afforded as an inseparable mixture.
B. O-PRENYLATED ACYLPHLOROGLUCINOLS
The isolation of five O-prenylated acylphloroglucinols, olympicins A–E (37–41) (aerial parts of H. olympicum)19 (Figure 5) and the synthesis of 37 using a simple four-step method were reported. Comparison of optical rotations for natural and synthetic olympicin A indicated the S configuration for the chiral center of acyl group in 37. Olympicin A (37) showed exceptional activities against methicillin-resistant and multidrug-resistant strains of S. aureus with MICs of 0.5–1 µg/mL, while 38–41 showed moderate activities (MICs 64–128 µg/mL). Furthermore, 37 exhibited antimicrobial activities against four Mycobacterium strains (M. smegmatis, M. fortuitum, M. phlei, and M. smegmatis). In contrast, 37 was not active against Gram-negative bacteria. Empetrifelixins A–D (42–45) (aerial parts of H. empetrifolium), antiproliferative PAPs possessing a limonene moiety linked via hydroxy groups of the acylphloroglucinol moieties, were isolated, together with a PAP with a bornane moiety, empetrikajaforin (46).8 Elegaphenone (47) (aerial parts of H. elegans) was assigned as a benzoylphloroglucinol with an O-geranyl group, and showed cytotoxicity against HD-MY-Z, K-562, and KE-37 cancer cell lines.20
C. PRENYLATED ACYLPHLOROGLUCINOLS WITH SPIRO-STRUCTURES
PAPs possessing unique spiro-structures with a geminal diprenyl group and a monoterpene moiety, tomoeones A–H (48–55) (Figure 6), were isolated from the aerial parts of H. ascyron.21
Tomoeones E (52) and F (53) demonstrated cytotoxicity against KB cells as well as multi-drug resistant cancer cell lines (KB-C2 and K562/Adr). Recently, benzoyl acylphloroglucinols with the related skeleton, hyperbeanols A–D (56–59) (aerial parts of H. beanii)22 have been reported. The structure including the relative stereochemistry of 56 was confirmed by a single crystal X-ray diffraction analysis, and 57 and 59 exhibited a modest cytotoxicity against K562 cells. The structures of spiro-PAPs, hyperielliptone HB (60) (heartwood of H. geminiflorum)23 and chipericumins C (61) and D (62) (roots of H. chinense),24 were elucidated. These compounds have a methyl and prenyl groups on the phloroglucinol moiety instead of a geminal diprenyl group in 48–59. Hyperielliptone HB (60) exhibited an inhibition of oxidative DNA damage and an inhibitory effect on xanthine oxidase. Chipericumins A (63) and B (64) (roots of H. chinense)24 are tetracyclic spiro-PAPs related biogenetically to 61 and 62. The relative configurations of 63 and 64 were deduced by analysis of the NOESY spectra. Phytochemical investigation of the aerial parts of H. sampsonii afforded a series of spiro-benzoylphloroglucinols, sampsonols A–F (65–70).25 Among them, 67–70 have unique tetracyclic structures with an ether linkage between the phloroglucinol and monoterpene moieties. Sampsonols A (65) and B (66) showed cytotoxicity against human tumor cell lines (MCF-7, HepG2, HT-29, and A549), while sampsonols C (67) and F (70) exhibited an inhibitory activity against LPS-induced NO production in RAW 264.7 macrophages.
D. POLYCYCLIC POLYPRENYLTED ACYLPHLOROGLUCINOLS
PAPs with highly oxygenated and densely substituted bicyclo[3.3.1]nonane-2,4,9-trione or bicyclo[3.2.1]octane-2,4,8-trione core are called as polycyclic polyprenylated acylphloroglucinols (PPAPs). Their fascinating chemical structures and intriguing biological activities have attracted widespread attention from organic synthesists and pharmacological endeavors.3,26-30 Hyperforin, a major constituent of H. perforatum (St. John’s Wort) responsible for its antidepressant effects, belongs to this class. This compound also showed antibacterial activity against Gram-positive bacteria and antitumoral activity in vivo.26,27 From 2006 to 2013, 23 PPAPs with bicyclo[3.3.1]nonane-2,4,9-trione core including 18-hydroxy-7-epi-clusianone (71) and 18-hydroxyclusianone (72) (roots of H. hypericoides),31 otogirinins D (73) and E (74)15 (aerial parts of H. erectum), sampsoniones N–P (75–77)32 (roots of H. sampsonii), uralodins B (78) and C (79) (aerial parts of H. henryi subsp. uraloides),33 hypercohins B–J (80–88) (aerial parts of H. cohaerens),34 hypersampsons H (89)35 and K (90)36 (fruits of H. samposonii), androforin A (91)37 (aerial parts of H. androsaemum), and prolifenones A (92) and B (93)16 (aerial parts of H. prolificum) were reported (Figures 7 and 8).
18-Hydroxy-7-epi-clusianone (71) and 18-hydroxyclusianone (72) possess a 3-hydroxybenzoyl group as the acyl moiety (Figure 7). A tetra- or di-hydrofuran ring adjacent to the phloroglucinol moiety is found in 73–86 and 88, while 74 and 80–82 have an additional tetrahydrofuran ring on the C10 side chains. On the other hand, 87, and 89–91 have a tetra- or di-hydropyran ring connected to the phloroglucinol moiety (Figure 8). Hypersampsone H (89) exhibited cytotoxicity against A549 cells. Prolifenones A (92) and B (93) are rare PPAPs with a geranyl group at C-16 and absence of an alkyl group at C-7.
Takaneones A–C (96–98)17 (aerial parts of H. sikokumontanum) (Figure 9) are tricyclic PPAPs with bicyclo[3.2.1]octane-2,4,8-trione core and C4 alkyl chain, and showed cytotoxicity against K562/Adr multi-drug resistant cancer cells. Recently, the isolation of an unusual PPAP possessing a bicyclo[5.3.1]hendecane-8,10,11-trione core accompanied by a six-membered ether ring, hypercohin A (99)38 (aerial parts H. cohaerens), was reported. The structure of 99 including the absolute configuration was elucidated by a single crystal X-ray diffractions analysis of its p-bromobenzoate ester. Hypercohin A (99) showed moderate inhibitory activity on acetylcholinesterase (AChE) and exhibited cytotoxicity against human cancer cell (HL-60, SMMC-7721, A549, MCF-7, and SW-80) and normal cell (Beas-2B) lines.
E. POLYCYCLIC POLYPRENYLATED ACYLPHLOROGLUCINOLS WITH CAGED STRUCTURES
Plants of Hypericaceae and Clusiaceae families (APG) produce a series of oxidized and polyprenylated benzophenone derivatives, some of which have complex caged structures with adamantane or homoadamantane skeletons. Several compounds belonging to this class showed a wide range of biological activity such as antimicrobial, antifungal, anticarcinogenic, and anti-HIV inhibitory activities.39 From 2006, caged PAPs, otogirinins A–C (100, 113, and 115) (aerial parts of H. erectum),15 sinaicinone (101) (aerial parts of H. sinaicum),40 hyperandrone A (103) (aerial parts of H. androsaemum),37 18-hydroxyhyperibone K (104) (roots of H. hypericoides),31 sampsonione Q (102),32 and peroxysampsones A (111) and B (112)41 (roots of H. sampsonii), hypersampsones G (105), I (106), J (107), and L (114) (fruits of H. sampsonii),35,36 and hypercohones A–C (108–110) (aerial parts of H. cohaerens)42 were reported (Figure 10). 100–104 are adamantane type caged PAPs. Among caged PAPs (105–115) with homoadamantae skeleton, 105–110 have an additional cyclopentane ring, whereas 111–113 possess an endo-peroxyde moiety. The possible biosynthetic pathway of hypersampson L (114), which has the structure with lack of an alkyl group at C-3, was discussed.
F. MEROTERPENOIDS
Several meroterpenoids have been reported from Hypericum plants, some of which have fascinating chemical structures and interesting biological activities. Investigation of the whole plants of H. yojiroanum resulted in the isolation of two biogenetically unique meroterpenoids with a γ-butyrolactone moiety, yojironins A (116) and B (117)13 (Figure 11), which seem to be composed of three isoprene units, two acetate units, and one 2-methylbutanoyl unit derived from isoleucine (Scheme 1). Yojironin A (116) exhibited cytotoxicity against murine lymphoma L1210 and human epidermoid carcinoma KB cells and showed antimicrobial activities against Aspergillus niger, Candida albicans, C. neoformans, T. mentagrophytes, S. aureus, and Bacillus subtilis.
Yezo’otogirins A–C (118–120) (Figure 11) are structurally unique tricyclic meroterpenoids isolated from the aerial parts of H. yezoense.43 The structure of 118, including octahydroindenofuran core, three tertiary methyl groups, two prenyl groups, and one 2-methylpropanoyl group, were revealed by analysis of the 1H-1H COSY and HMBC spectra, taking the molecular formula elucidated by the HRESIMS into consideration (Figure 12). Interpretation of the NOESY spectrum of 118 suggested the β-orientations for the substituents at C-2, C-3, and C-4. The absolute stereochemistry of 118 was elucidated based on analysis of the CD spectrum. Similarly, the structures of 119 and 120 were assigned. The bioinspired protective group-free total synthesis of (±)-yezo’otogirin C was achieved from 3-methyl-4-prenylcylohex-2-enone by Lee et al.44
Three meroterpenoids with spiro-lactone structures, hyperolactones A–C (121–123), and one related lactone, hyperolactone D (124), were isolated from the leaves of H. chinense (Figure 13).45 Biosynthetic pathway of 121–124 was also proposed, where 121–124 may be biosynthesized from isopentenyl phosphate and polyketide. Hyperolactone D related meroterpenoids, 4-hydroxyhyperolatone D (125) and 5,6-dihydrohyperolactone D (126), were reported from the same species.46 The absolute stereochemistries of hyperolactones A (121) and B (122) were confirmed by the total syntheses.47-49 The total syntheses of hyperolactone C (123) were also reported by several groups.48,50-56 Recently, biomimetic syntheses of hyperolactone D (124) and 4-hydroxyhyperolactone D (126) has been reported.55
Together with 125 and 126, structurally unique meroterpenoids, biyouyanagins A (127)57 and B (128)46 (Figure 13), which have hybrid structures of 123 and a sesquiterpene (ent-zingiberene), were isolated from the leaves of H. chinense. Biyouyanagin A (127) exhibited an anti-HIV activity and showed an inhibitory activity against LPS-induced production of IL-10, IL-12, and TNF-α. The total syntheses of 127 and 128 proceeded by Nicolaou et al. through cascade sequences that produced enantiomerically pure building blocks ent-zingiberene and 123 and featured a novel [2+2] photoinduced cycloaddition reaction, resulting in the revision of the stereochemistries of 127 and 128.51,54,58 Xie et al. also achieved the total synthesis of 127.53 Recently, the library of synthesized analogues of 127 was subjected to biological screening, aiming to search lead compounds for antiviral and anti-inflammatory agents.59
Six meroterpenoids, biyoulactones A–E (129–133) (roots of H. chinense)60,61 and biyouyanagiol (134) (stems of H. chinense),46 were isolated (Figure 14). Biyoulactones A–C (129–131) are pentacyclic meroterpenoids with a unique structure containing C–C bonded bi- and tri-cyclic γ-lactone moieties. The gross structures of three partial structures (units A–C) in 129 were elucidated based on analysis of the 2D NMR spectra (Figure 15). A single crystal X-ray diffraction analysis of 129 revealed the connectivities among units A–C as well as the absolute stereochemistry of 129.
Biyoulactones D (132) and E (133) are meroterpenoids possessing an octahydroindene ring, a γ-butyrolactone ring, and a β-diketone moiety. The structures of 132 and 133 including the relative configurations were assigned based on spectroscopic data and conformational analysis. The octahydroindene moiety is found in 129–134 in common, which is also seen in PAPs 61–64 (Figure 6) isolated from the same origin. These meroterpenoids (129–134) and PAPs (61–64) are presumed to be biogenetically related to PAPs, chinesins I or II,62 major constituents for the flowers of H. chinense.
A meroterpenoid with a novel skeleton containing an oxepane ring, hypertricone (135), was reported from the leaves of H. geminiflorum63 (Figure 16). Examination of the aerial parts of H. perforatum yielded a meroterpenoid, preforatumone (136).64 A plausible biosynthetic route of 136 from hyperforin involving a Baeyer-Villiger ring cleavage and pinacol rearrangement was proposed. Meroterpenoids, hyperscabrins A–C (137–139), were reported as constituents of the aerial parts of H. scabrum.65 The absolute configurations of 137–139 were elucidated by study of the CD spectra.
CONCLUSIONS
Studies for the isolation and structure elucidation of prenylated acylphloroglucinols (PAPs) isolated from Hypericum plants from 2006 to 2013 were reviewed. A part of PAPs were found to show interesting biological activity, whereas many of them have not been fully evaluated for their biological activities. Meroterpenoids from Hypericum plants were also summarized, whose unique chemical structures and intriguing biological activities will attract attention as new drug leads as well as challenging target of total synthesis. Though meroterpenoids from Hypericum plants have a wide variety of chemical structures, their biosynthesis pathway remains largely unsolved. Further efforts at understanding the properties of PAPs and meroterpenoids from Hypericum plants as well as phytochemical investigations of this genus are therefore needed.
References
1. P. Avato, 'Studies in Natural Products Chemistry,' Vol. 30, ed. by Atta-ur-Rahman, Elsevier Sience B.V., Amsterdam, 2005, pp. 603-634.
2. M. Wurglics and M. Schubert-Zsilavecz, Clin. Pharmacokinet., 2006, 45, 449. CrossRef
3. R. Ciochina and R. B. Grossman, Chem. Rev., 2006, 106, 3963. CrossRef
4. I. P. Singh and S. B. Bharate, Nat. Prod. Rep., 2006, 23, 558. CrossRef
5. K. Manning, E. Petrunak, M. Lebo, A. González-Sarrías, N. P. Seeram, and G. E. Henry, Phytochemistry, 2011, 72, 662. CrossRef
6. W. Wang, Y. H. Zeng, K. Osman, K. Shinde, M. Rahman, S. Gibbons, and Q. Mu, J. Nat. Prod., 2010, 73, 1815. CrossRef
7. . CrossRef
8. S. Schmidt, G. Jürgenliemk, H. Skaltsa, and J. Heilmann, Phytochemistry, 2012, 77, 218. CrossRef
9. A. P. M. Bernardi, A. B. F. Ferraz, D. V. Albring, S. A. L. Bordignon, J. Schripsema, R. Bridi, C. S. Dutra-Filho, A. T. Henriques, and G. L. von Poser, J. Nat. Prod., 2005, 68, 784. CrossRef
10. S. Schmidt, G. Jürgenliemk, T. J. Schmidt, H. Skaltsa, and J. Heilmann, J. Nat. Prod., 2012, 75, 1697. CrossRef
11. N. Tanaka, T. Kubota, H. Ishiyama, A. Araki, Y. Kashiwada, Y. Takaishi, Y. Mikami, and J. Kobayashi, Bioorg. Med. Chem., 2008, 16, 5619. CrossRef
12. N. Tanaka, M. Otani, Y. Kashiwada, Y. Takaishi, A. Shibazaki, T. Gonoi, M. Shiro, and J. Kobayashi, Bioorg. Med. Chem. Lett., 2010, 20, 4451. CrossRef
13. T. Mamemura, N. Tanaka, A. Shibazaki, T. Gonoi, and J. Kobayashi, Tetrahedron Lett., 2011, 52, 3575. CrossRef
14. N. Tanaka, T. Mamemura, A. Shibazaki, T. Gonoi, and J. Kobayashi, Bioorg. Med. Chem. Lett., 2011, 21, 5393.
15. Y. Ishida, O. Shirota, S. Sekita, K. Someya, F. Tokita, T. Nakane, and M. Kuroyanagi, Chem. Pharm. Bull., 2010, 58, 336. CrossRef
16. G. E. Henry, S. Raithore, Y. Zhang, B. Jayaprakasam, M. G. Nair, D. Heber, and N. P. Seeram, J. Nat. Prod., 2006, 69, 1645.
17. N. Tanaka, Y. Kashiwada, M. Sekiya, Y. Ikeshiro, and Y. Takaishi, Tetrahedron Lett., 2008, 49, 2799. CrossRef
18. N. Tanaka, T. Kubota, H. Ishiyama, Y. Kashiwada, Y. Takaishi, J. Ito, Y, Mikami, M. Shiro, and J. Kobayashi, Heterocycles, 2009, 79, 917.
19. W. K. P. Shiu, M. M. Rahman, J. Curry, P. Stapleton, M. Zloh, J. P. Malkinson, and S. Gibbons, J. Nat. Prod., 2012, 75, 336. CrossRef
20. P. T. Nedialkov, D. Zheleva-Dimitrova, G. Momekov, K. Karlov, U. Girreser, and G. M. Kitanov, Nat. Prod. Res., 2011, 25, 1743. CrossRef
21. W. Hashida, N. Tanaka, Y. Kashiwada, M. Sekiya, Y. Ikeshiro, and Y. Takaishi, Phytochemistry, 2008, 69, 2225. CrossRef
22. X.-Q. Chen, Y. Li, K.-Z. Li, L.-Y. Peng, J. He, K. Wang, Z.-H. Pan, X. Cheng, M.-M. Li, Q.-S. Zhao, and G. Xu, Chem. Pharm. Bull., 2011, 59, 1250. CrossRef
23. C.-C. Wu, M.-H. Yen, S.-C. Yang, and C.-N. Lin, J. Nat. Prod., 2008, 71, 1027. CrossRef
24. S. Abe, N. Tanaka, and J. Kobayashi, J. Nat. Prod., 2012, 75, 484. CrossRef
25. W.-B. Xin, X.-H. Man, C.-J. Zheng, M. Jia, Y.-P. Jiang, X.-X. Zhao, G.-L. Jin, Z.-J, Mao, H.-Q. Huang, and L.-P. Qin, Fitoterapia, 2012, 83, 1540. CrossRef
26. M. A. Medina, B. Martínez-Poveda, M. I. Amores-Sánchez, and A. R. Quesada, Life Sci., 2006, 79, 105. CrossRef
27. L. Beerhues, Phytochemistry, 2006, 67, 2201. CrossRef
28. I. P. Singh, J. Sidana, S. B. Bharate, and W. J. Foley, Nat. Prod. Rep., 2010, 27, 393. CrossRef
29. J. T. Njardarson, Tetrahedron, 2011, 67, 7631. CrossRef
30. J.-A. Richard, R. H. Pouwer, and D. Y.-K. Chen, Angew. Chem. Int. Ed., 2012, 51, 4536. CrossRef
31. O. E. Christian, S. McLearn, W. F. Reynolds, and H. Jacobs, Nat. Prod. Commun., 2008, 3, 1781.
32. Z. Y. Xiao, Q. Mu, W. K. P. Shiu, Y. H. Zeng, and S. Gibbons, J. Nat. Prod., 2007, 70, 1779. CrossRef
33. X.-Q. Chen, Y. Li, X. Cheng, K. Wang, J. He, Z.-H. Pan, M.-M. Li, L.-Y. Peng, G. Xu, and Q.-S. Zhao, Chem. Biodiversity, 2010, 7, 196. CrossRef
34. X. Liu, X.-W. Yang, C.-Q. Chen, C.-Y. Wu, J.-J. Zhang, J.-Z. Ma, H. Wang, L.-X. Yang, and G. Xu, J. Nat. Prod., 2013, 76, 1612. CrossRef
35. Y. H. Zeng, Q. Mu, Z. Y. Xiao, Y. Xu, M. M. Rahman, and S. Gibbons, Chem. Lett., 2009, 38, 440. CrossRef
36. Y.-H. Zeng, K. Osman, Z.-Y. Xiao, S. Gibbons, and Q. Mu, Phytochemistry Lett., 2012, 5, 200. CrossRef
37. K. Wang, Y.-Y. Wang, X. Gao, X.-Q. Chen, L.-Y. Peng, Y. Li, G. Xu, and Q.-S. Zhao, Chem. Biodiversity, 2012, 9, 1213. CrossRef
38. X.-W. Yang, X. Deng, X. Liu, C.-Y. Wu, X.-N. Li, B. Wu, H.-R. Luo, Y. Li, H.-X. Xu, Q.-S. Zhao, and G. Xu, Chem. Commun., 2012, 48, 5998. CrossRef
39. O. Cuesta-Rubio, A. L. Piccinelli, and L. Rastrelli, 'Studies in Natural Products Chemistry,' Vol. 32, ed. by Atta-ur-Rahman, Elsevier Sience B.V., Amsterdam, 2005, pp. 671-720.
40. T. Rezanka and K. Sigler, Phytochemistry, 2007, 68, 1272. CrossRef
41. Z. Y. Xiao, Y. H. Zeng, Q. Mu, W. K. P. Shiu, and S. Gibbons, Chem. Biodiversity, 2010, 7, 953. CrossRef
42. X. Liu, X.-W. Yang, C.-Q. Chen, C.-Y. Wu, J.-J. Zhang, J.-Z. Ma, H. Wang, Q.-S. Zhao, L.-X. Yang, and G. Xu, Nat. Prod. Bioprospect., 2013, 3, 233. CrossRef
43. N. Tanaka, Y. Kakuguchi, H. Ishiyama, T. Kubota, and J. Kobayashi, Tetrahedron Lett., 2009, 50, 4747. CrossRef
44. S. He, W. Yang, L. Zhu, G. Du, and C.-S. Lee, Org. Lett., 2014, 16, 496. CrossRef
45. Y. Aramaki, K. Chiba, and M. Tada, Phytochemistry, 1995, 38, 1419. CrossRef
46. N. Tanaka, Y. Kashiwada, S. Y. Kim, W. Hashida, M. Sekiya, Y. Ikeshiro, and Y. Takaishi, J. Nat. Prod., 2009, 72, 1447. CrossRef
47. D. Ichinari, T. Ueki, K. Yoshihara, and T. Kinoshita, Chem. Commun., 1997, 1743. CrossRef
48. T. Ueki, M. Doe, R. Tanaka, Y. Morimoto, K. Yoshihara, and T. Kinoshita, J. Heterocycl. Chem., 2001, 38, 165. CrossRef
49. T. Ueki, D. Ichinari, K. Yoshihara, Y. Morimoto, and T. Kinoshita, Tetrahedron Lett., 1998, 39, 667. CrossRef
50. G. A. Kraus and J. Wei, J. Nat. Prod., 2004, 67, 1039. CrossRef
51. K. C. Nicolaou, T. R. Wu, D. Sarlah, D. M. Shaw, E. Rowcliffe, and D. R. Burton, J. Am. Chem. Soc., 2008, 130, 11114. CrossRef
52. D. M. Hodgson, D. Angrish, S. P. Erickson, J. Kloesges, and C. H. Lee, Org. Lett., 2008, 10, 5553. CrossRef
53. C. Du, L. Li, Y. Li, and Z. Xie, Angew. Chem. Int. Ed., 2009, 48, 7853. CrossRef
54. K. C. Nicolaou, D. Sarlah, and D. M. Shaw, Angew. Chem. Int. Ed., 2007, 46, 4708. CrossRef
55. Y. Wu, C. Du, C. Hu, Y. Li, and Z. Xie, J. Org. Chem., 2011, 76, 4075. CrossRef
56. D. M. Hodgson and S. Man, Chem. Eur. J., 2011, 17, 9731. CrossRef
57. N. Tanaka, M. Okasaka, Y. Ishimaru, Y. Takaishi, M. Sato, M. Okamoto, T. Oshikawa, S. U. Ahmed, L. M. Consentino, and K.-H. Lee, Org. Lett., 2005, 7, 2997. CrossRef
58. K. C. Nicolaou, S. Sanchini, T. R. Wu, and D. Sarlah, Chem. Eur. J., 2010, 16, 7678. CrossRef
59. K. C. Nicolaou, S. Sanchini, D. Sarlah, G. Lu, T. R. Wu, D. K. Nomura, B. F. Cravatt, B. Cubitt, J. C. de la Torre, A. J. Hessell, and D. R. Burton, Proc. Natl. Acad. Sci. USA, 2011, 108, 6715. CrossRef
60. N. Tanaka, S. Abe, K. Hasegawa, M. Shiro, and J. Kobayashi, Org. Lett., 2011, 13, 5488. CrossRef
61. N. Tanaka, S. Abe, and J. Kobayashi, Tetrahedron Lett., 2012, 53, 1507. CrossRef
62. M. Nagai and M. Tada, Chem. Lett., 1987, 16, 1337. CrossRef
63. J.-R. Weng, M.-I. Chung, M.-H. Yen, C.-N. Lin, and R.-R. Wu, Helv. Chim. Acta, 2001, 84, 1976. CrossRef
64. J. Wu, X.-F. Cheng, L. J. Harrison, S.-H. Goh, and K.-Y. Sim, Tetrahedron Lett., 2004, 45, 9657. CrossRef
65. J. Ma, T.-F. Ji, J.-B. Yang, A.-G. Wang, and Y.-L. Su, J. Asian Nat. Prod. Res., 2012, 14, 508. CrossRef