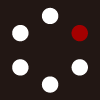
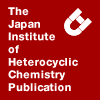
HETEROCYCLES
An International Journal for Reviews and Communications in Heterocyclic ChemistryWeb Edition ISSN: 1881-0942
Published online by The Japan Institute of Heterocyclic Chemistry
e-Journal
Full Text HTML
Received, 3rd June, 2014, Accepted, 11th July, 2014, Published online, 23rd July, 2014.
DOI: 10.3987/COM-14-S(K)35
■ Design of Aza-Polyquinanes via Fischer Indole Cyclization under Green Conditions
Sambasivarao Kotha* and Ajay Kumar Chinnam
Department of Chemistry, Indian Institute of Technology Bombay, Powai, Mumbai - 400076, India
Abstract
We have demonstrated a simple and an efficient synthetic route for the synthesis of aza-polyquinane derivatives involving Fischer indole cyclization as a key step under low melting mixture conditions.Synthesis and design of polyquinanes is actively pursued during last three decades.1 Isolation of various natural products and activity in theoretically interesting non-natural products containing quinane systems has fueled the growth of polyquinane area. Recently, various hetero-atom containing polyquinanes appeared in the literature as useful targets.2 König and co-workers reported low melting mixtures as a green reaction media for promoting the Fischer indole cyclization. These low melting mixture conditions (tartaric acid and dimethyl urea) are considered as green because of mild reaction conditions, ease of handling, simple work up and recycling ability aspects.3 In connection with our interest in utilizing a readily available building blocks to generate “drug like” molecules in diversity oriented fashion, we studied Fischer indole cyclization of Weiss–Cook diones4a in low melting mixtures. To this end, tricyclic [4.3.3]propellane dione 1 was shown to undergo two-fold Fischer indole cyclization to generate bis-indole derivatives. Latter, this methodology has been extended to the synthesis of various other propellane derivatives (Scheme 1).4b,4c
To expand the scope of this methodology here we used intricate polyquinane derivatives to generate polycyclic bis-indole derivatives. Pentaquinanes 12 and 13 used in the present study are easily available by a five step sequence from a readily available starting materials such as cyclopentadiene 5 and 1,4-benzoquinone 6. These diones 12 and 13 are attractive targets for further synthetic exploitation due to their easy accessibility and stable nature.5 Some time back Fischer indole cyclization, Friedlander annulation and other benzanulation reactions were studied to design novel molecular entities.6-9
Pentaquinane derivative 12 synthesis used here start with Diels–Alder reaction. Thus, cyclopentadiene 5 on Diels–Alder reaction with 1,4-naphthoquinone 6 gave the Diels–Alder adduct 7, which on [2+2] photocycloaddition delivered the hexacyclic propellane derivative 8. Latter, the diketone 8 was subjected to reduction in the presence of Zn-AcOH to produce an annulated trishomocubane derivative 9. The cubane derivative 9 was refluxed under NaOMe-MeOH conditions to generate a mixture of rearranged diones 10 and 11. Latter, the diones 10 and 11 were subjected to succinyl C-C bond cleavage under Na-K alloy-Me3SiCl conditions to furnish the pentacyclic diones 12 and 13 respectively (Scheme 2).
Next, the pentacyclic dione 12 was treated with two equivalents of 1-methyl-1-phenylhydrazine 14 in the presence of a low melting mixture of L-(+)-tartaric acid (TA) and dimethyl urea (DMU) to generate two-fold Fischer indole cyclization product 15. Hydrogenation of 15 in the presence of 10 mol% Pd/C in EtOAc gave the saturated diindole 17. In another route pentaquinane derivative 12 was hydrogenated and then subjected to two-fold Fischer indole cyclization to deliver the bis aza-polyquinane derivative 17. The product 17 obtained by this procedure was found to be identical to that of the compound obtained by the earlier route (Scheme 3).
Later, methoxy substituted pentacyclic dione 13 was also subjected to two-fold Fischer indole cyclization in low melting mixture with two equivalents of 1-methyl-1-phenylhydrazine 14 is expected to deliver the bis-indole derivative 19. Surprisingly, mono-indole derivative 18 was obtained in 95% yield (Scheme 4).
It appears that only one side pentacyclic dione had undergone Fischer indole cyclization due to the presence of the methoxy group creating the steric barrier. The structure of 18 was unambiguously established by single crystal X-ray diffraction studies (Figure 1).10
In summary, we have developed a new and simple synthetic route towards the synthesis of aza-polyquinane derivatives containing up to nine rings in six step sequence starting with cyclopentadiene and 1,4-naphthaquinanone by using Fischer indole cyclization as a key step. Since macrocyclic indole derivatives are implicated in medicinal chemistry, our results are likely to draw the attention of medicinal chemists and drug discovery persons.
EXPERIMENTAL
NMR spectra were recorded at rt on 400 MHz Bruker NMR spectrometer in CDCl3 solution. Coupling constants (J values) are given in Hertz (Hz). Melting points were recorded with Buchi melting point apparatus. Infrared (IR) spectra were recorded Nicolet Impact-400 FT IR spectrometer in KBr. The high-resolution mass measurements were carried out using a Micromass Q-ToF spectrometer. Analytical thin layer chromatography (TLC) was performed on (10 × 5 cm) glass plates coated with Acme’s silica gel GF 254 (containing 13% calcium sulfate as a binder). Chromatography was performed using Acme’s silica gel (100-200 mesh) using double spray bellows for application of pressure and the column is eluted with EtOAc/petroleum ether mixture. The organic solvents used in this study were dried over appropriate drying agents and distilled prior to use.
General procedure for the Fischer indole cyclization.
In a typical experiment, 1.5 g of L-(+)-tartaric acid- N,N′−dimethylurea (30:70) mixture was heated to 70 oC to obtain a clear melt. To this melt, 2 mmol of 1-methyl-1-phenyl hydrazine and 1 mmol of diketone were added at 70 oC. At the conclusion of the reaction (TLC monitoring), the reaction mixture was quenched by adding water while still hot. The reaction mixture was cooled to room temperature and the solid that separated was filtered and washed with water (2 × 5 mL). The crude product was dried under vacuum and then it was purified by silica gel column chromatography.
Two fold Fischer indole cyclization of 15
The pentacyclic dione 12 (100 mg, 0.44 mmol) reacted with 1-methyl-1-phenylhydrazine (118 mg, 0.96 mmol) in the presence of low melting mixture by following the general procedure. At the conclusion of the reaction (TLC monitoring), the reaction mixture was workup according to the general procedure. The crude product obtained was purified by column chromatography on a silica gel (EtOAc/petroleum ether, 10:90) to provide diindole derivative 15 (134 mg, 76%) as a colorless solid.
Rf = 0.38 (Silica gel, 10% EtOAc/petroleum ether).
mp 152-153 oC.
IR (KBr): νmax 3019, 2952, 2855, 1406, 1216, 1155 cm-1.
1H NMR (400 MHz, CDCl3) δ = 2.26-2.31 (m, 1H), 2.35-2.42 (m, 1H), 2.51-2.57 (m, 1H), 2.93-3.00 (m, 1H), 3.02-3.06 (m, 1H), 3.46 (s, 3H), 3.56 (s, 3H), 3.71-3.78 (m, 2H), 3.82-3.92 (m, 2H), 4.19-4.26 (m, 1H), 5.82-5.83 (d, J = 6.28 Hz, 2H), 6.82-6.88 (m, 2H), 6.91-6.93 (m, 2H), 6.96-6.99 (m, 2H), 7.27-7.29 (m, 2H).
13C NMR (100 MHz, CDCl3) δ = 29.54, 30.50, 36.64, 40.34, 44.94, 45.87, 52.88, 52.99, 62.38, 69.21, 71.07, 108.91, 108.97, 118.21, 118.24, 118.72, 119.85, 119.97, 121.76, 122.30, 123.40, 123.60, 129.14, 137.19, 141.96, 142.20, 145.49, 146.28
HRMS (ESI): m/z [M + H]+ calcd for C29H27N2: 403.2169; found: 403.2169.
Synthesis of Compound 17
Method A
To a solution of diindole 15 (50 mg, 0.12 mmol) in dry EtOAc (10 mL), 10% Pd/C (10 mg, 0.09 mmol) was added and the reaction mixture was stirred at room temperature under H2 atmosphere (1 atm) for 4 h. At the end of the reaction (TLC monitoring), the reaction mixture was filtered through a pad of celite and washed with EtOAc (20 mL). Evaporation of the solvent in vacuo gave the crude product. Further purification by silica-gel column chromatography (5% EtOAc/petroleum ether) afforded the hydrogenated product 17 (46 mg, 92%) as a colorless solid.
Method B
Hydrogenation of Compound 12
To a solution of dione 12 (50 mg, 0.22 mmol) in dry EtOAc (10 mL), 10% Pd/C (10 mg, 0.09 mmol) was added and the reaction mixture was stirred at room temperature under H2 atmosphere (1 atm) for 4 h. At the end of the reaction (TLC monitoring), the reaction mixture was filtered through a pad of celite and washed with ethyl acetate (20 mL). Evaporation of the solvent in vacuo gave the crude product. Further purification by silica-gel column chromatography (5% EtOAc/petroleum ether) afforded the hydrogenated product 16 (47 mg, 93%) as a colorless solid.
Rf = 0.37 (Silica gel, 10% EtOAc/petroleum ether).
mp 94-96 oC.
IR (KBr): νmax 3020, 2928, 2855, 1730, 1216, 1045 cm-1.
1H NMR (400 MHz, CDCl3) δ = 0.76 (q, J = 11.89 Hz, 1H), 1.57-1.71 (m, 3H), 1.75-1.85 (m, 2H), 1.87-1.96 (m, 1H), 2.14-2.21 (m, 1H), 2.23-2.33 (m, 2H), 2.28-2.54 (m, 4H), 2.66-2.77 (m, 2H), 3.08 (t, J = 8.33 Hz, 1H), 3.39-3.45 (q, J = 8.44 Hz, 1H).
13C NMR (100 MHz, CDCl3) δ = 25.85, 35.67, 38.98, 39.62, 41.00, 41.37, 44.98, 45.90, 54.58, 57.16, 61.25, 62.37, 70.37, 221.06, 223.58.
HRMS (ESI): m/z [M+H]+ calcd for C15H19O2: 231.1380; found: 231.1375.
Fischer indole cyclization of 16
The pentacyclic dione 16 (50 mg, 0.22 mmol) reacted with 1-methyl-1-phenylhydrazine (59 mg, 0.48 mmol) in the presence of low melting mixture by following the general procedure. At the conclusion of the reaction (TLC monitoring), the reaction mixture was workup by according to the general procedure. The crude product obtained was purified by column chromatography on silica gel (EtOAc/petroleum ether, 10:90) to provide diindole derivative 17 (83 mg, 94%) as a colourless solid.
Rf = 0.40 (Silica gel, 10% EtOAc/petroleum ether).
mp 208-210 oC.
IR (KBr): νmax 3165, 3021, 2942, 2293, 2254, 1442, 1374, 1219, 1038 cm-1.
1H NMR (400 MHz, CDCl3) δ = 1.57-1.61 (m, 1H), 1.76-1.80 (m, 1H), 1.83-1.93 (m, 1H), 1.96-2.08 (m, 3H), 2.11-2.19 (m, 1H), 2.72-2.75 (m, 1H), 2.88-2.91 (m, 1H), 3.39 (s, 3H), 3.44 (s, 3H), 3.46-3.48 (m, 1H), 3.66-3.69 (m, 3H), 3.96-4.01 (m, 1H), 6.60-6.67 (m, 6H), 7.13-7.19 (m, 2H).
13C NMR (100 MHz, CDCl3) δ = 25.16, 29.94, 30.39, 34.15, 34.54, 38.71, 43.20, 44.33, 50.68, 51.94, 60.95, 63.15, 70.85, 108.38, 108.46, 117.87, 118.08, 118.46, 119.52, 121.90, 121.96, 123.41, 123.45, 141.94, 142.19, 144.98, 146.32.
HRMS (ESI): m/z [M + Na]+ calcd for C29H28N2Na: 427.2149; found: 427.2145.
Compound 18
The pentacyclic dione 13 (50 mg, 0.19 mmol) reacted with 1-methyl-1-phenylhydrazine (52 mg, 0.42 mmol) in the presence of low melting mixture by following the general procedure. At the conclusion of the reaction (TLC monitoring), the reaction mixture was workup by according to the general procedure. The crude product obtained was purified by column chromatography on silica gel (EtOAc/petroleum ether, 10:90) to provide indole derivative 18 (63 mg, 95%) as a colorless solid.
Rf = 0.36 (Silica gel, 10% EtOAc/petroleum ether).
mp 196-198 oC.
IR (KBr): νmax 3006, 2929, 2874, 2826, 1719, 1466, 1174, 1104 cm-1.
1H NMR (400 MHz, CDCl3) δ = 1.50-1.57 (dd, J1 = 7.65 Hz, J2 = 2.41 Hz, 1H), 1.58-1.67 (m, 1H), 1.75-1.83 (m, 1H), 2.04-2.10 (m, 1H), 2.12-2.19 (m, 1H), 2.20-2.28 (m, 2H), 2.44-2.46 (d, J = 13.21 Hz, 1H), 2.80-2.90 (m, 1H), 3.04-3.05 (m, 1H), 3.18 (s, 3H), 3.49-3.56 (m, 2H), 3.58 (s, 3H), 3.77-3.80 (t, J = 7.01 Hz, 1H), 4.01-4.04 (t, J = 7.90 Hz, 1H), 4.07-4.12 (m, 1H), 7.06-7.09 (m, 1H), 7.13-7.18 (m, 1H), 7.20-7.22 (m, 1H), 7.47-7.49 (d, J = 7.80 Hz, 1H).
13C NMR (100 MHz, CDCl3) δ = 28.53, 30.41, 31.08, 37.72, 39.35, 43.56, 45.60, 50.98, 53.06, 58.14, 58.29, 63.32, 73.57, 88.22, 109.84, 118.49, 119.16, 120.92, 121.47, 123.15, 142.90, 144.83, 225.35.
HRMS (ESI): m/z [M + Na]+ calcd for C23H25NNaO2: 370.1777; found: 370.1777.
ACKNOWLEDGMENT
We thank CSIR and Department of Science and Technology (DST), New Delhi for the financial support. SK thanks DST for the award of a J. C. Bose fellowship. AKC thanks University Grant Commission, New Delhi for the award of a research fellowship.
References
1. (a) L. A. Paquette, Top. Curr Chem., 1979, 79; (b) L. A. Paquette, Top. Curr Chem., 1984, 119; (c) M. Ramaiah, Synthesis, 1984, 529; CrossRef (d) L. A. Paquette and A. M. Doherty, Polyquinane Chemistry, Synthesis and Reactions, (Springer-Velrag, New York) 1987; CrossRef (e) G. Mehta and A. Srikrishna, Chem. Rev., 1997, 97, 671; CrossRef (f) L. A. Paquette and J. Doyon, J. Org. Chem., 1997, 62, 1723; CrossRef (g) C. A. Dvorak, C. Dufour, S. Iwasa, and V. H. Rawal, J. Org. Chem., 1998, 63, 5302; CrossRef (h) P. A. Wender and T. M. Dore, Tetrahedron Lett., 1998, 39, 8589; CrossRef (i) V. Singh and B. Thomas, Tetrahedron, 1998, 54, 3647. CrossRef
2. (a) M. Toyota, Y. Nishikawa, K. Motoki, N. Yoshida, and K. Fukumoto, Tetrahedron, 1993, 49, 11189; CrossRef (b) K. Fukumoto and S. Toyoda, Jpn. Patent 1995 95, 179, 449 (Chem. Abstr., 1995, 123, 257083d); (c) K. Masuya, K. Domon, K. Tanino, and I. Kuwajima, J. Am. Chem. Soc., 1998, 120, 1724; CrossRef (d) M. Lautens and J. Blackwell, Synthesis, 1998, 537; CrossRef (e) V. Krishnamurthy and V. H. Rawal, J. Braz. Chem. Soc., 1998, 9, 341; CrossRef (f) V. Singh, B. C. Sahu, and S. M. Mobin, Synlett, 2008, 1222; CrossRef (g) A. Bandaru and K. P. Kaliappan, Synlett, 2012, 23, 1473; CrossRef (h) K. Ramakrishna and K. P. Kaliappan, Synlett, 2011, 2580; (i) R. Datta, S. Bose, P. B. Viththlbhai, and S. Ghosh, Tetrahedron Lett., 2014, 55, 3538. CrossRef
3. S. Gore, S. Baskaran, and B, König, Org. Lett., 2012, 14, 4568. CrossRef
4. (a) X. Fu and J. M. Cook, Aldrichimica Acta, 1992, 25, 43; (b) S. Kotha, A. K. Chinnam, and A. Tiwari, Beilstein J. Org. Chem., 2013, 9, 2709; CrossRef (c) S. Kotha and A. K. Chinnam, Synthesis, 2014, 46, 301. CrossRef
5. (a) G. Mehta, S. Kotha, M. M. Bhadabhade, and K. Venkatesan, J. Chem. Soc., Chem. Commun., 1981, 755; CrossRef (b) G. Mehta and S. Kotha, J. Org. Chem., 1985, 50, 5537. CrossRef
6. (a) G. Mehta, A. Srikrishna, A. V. Reddy, and M. S. Nair, Tetrahedron, 1981, 37, 4559; CrossRef (b) W. Adam, E. Gogonas, and L. P. Hadjiarapoglou, J. Org. Chem., 2003, 68, 9155; CrossRef (c) C.-C. Liao, Pure. Appl. Chem., 2005, 77, 1221; (d) A. Srikrishna and D. H. Dethe, Indian J. Chem., 2011, 50B, 1092.
7. (a) G. Mehta and C. Prabhakar, Tetrahedron Lett., 1989, 30, 6895; CrossRef (b) G. Mehta, C. Prabhakar, N. Krishnamurthy, and M. S. Nair, Synth. Commun., 1990, 20, 3467; CrossRef (c) G. Mehta and C. Prabhakar, J. Org. Chem., 1995, 60, 4638. CrossRef
8. G. Mehta and C. Prabhakar, Indian J. Chem., 1995, 34B, 267.
9. R. P. Thummel, Tetrahedron, 1991, 47, 6851. CrossRef
10. CCDC-1005894 contains the supplementary crystallographic data for this paper. These data can be obtained free of charge from The Cambridge Crystallographic Data Centre via www.ccdc.cam.ac.uk/data request/cif. Crystal data: (18): C23H25NO2, M+ = 347, monoclinic, space group P 21/n, unit cells dimensions a = 9.2592 (9) A° α = 90 deg, b = 9.559 (11), c = 19.72 1 (2) A° γ = 90 deg, β = 91.251 (9)°, Z = 4, Dc = 1.323 g cm-3, F(000) = 744, λ (Mo-Kα) = 0.71 cm-1; 1812 observed reflections [|F| ≥ 3σ(|F|), 2Ɵ ≤ 50°] were measured on high resolution single crystal X-ray diffraction data at 293 K and the structure was solved by MULTAN 78 and refined by full-matrix least-square method to R 0.064. The figure shows a prospective view of the molecule.