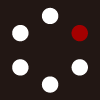
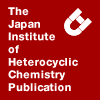
HETEROCYCLES
An International Journal for Reviews and Communications in Heterocyclic ChemistryWeb Edition ISSN: 1881-0942
Published online by The Japan Institute of Heterocyclic Chemistry
e-Journal
Full Text HTML
Received, 15th October, 2008, Accepted, 17th November, 2008, Published online, 18th November, 2008.
DOI: 10.3987/COM-08-S(F)121
■ Synthesis of New Chiral 5,6,7,8-Tetrahydrotetrazolo[1,5-a]pyrazines from α-Amino Acid Derivatives Following “Click“ Chemistry
Debendra K. Mohapatra,* Pradip K. Maity, Ravindra V. Ghorpade, and Mukund K. Gurjar
Division of Organic Chemistry, National Chemical Laboratory, Pashan Road, Pune 411 008, India
Abstract
An efficient and practical synthesis of new chiral fused tetrazoles have been synthesized following [3+2] cycloaddition reaction starting from α-amino acid derivatives.Tetrazole ring system are important features in biological systems, natural products, and drugs.1 These nitrogen rich systems are frequently used as a metabolically stable isosteric replacement for the carboxylic acid moiety and as a cis peptide bond mimetic.2 Tetrazoles have also been used as precursors to other heterocycles.3 For instance, Losartan (1) is a Angiotensin II antagonist and commonly used for treatment of hypertension.4 Tetrazole 3 has also been found to possess binding affinity to benzodiazepine receptors.5 Pentylentetrazole (PTZ) (2) has the opposite effect compared to 3 and is extensively used in models for anxiety, mediated by its unspecific interaction with a number of receptors in the CNS.6 Mannose mimetics 4 and 5 have been reported to be inhibitors of α-mannosidase (Figure 1).7
A variety of synthetic tetrazole containing biologically active substances are described in the current literature, such as glycin-tetrazole, modified S-alkyl-GSH analogues, α-methylene tetrazole based peptidomimetic HIV protease inhibitors, endothelin-converting enzyme (ECE-1), neutral endopeptidase 24.11 (NEP 24.11), non peptidic inhibitors and area-based inhibitors of glutamate carboxypeptidase II (GCP II). They have been investigated for medicinal applications in area as diverse as antibacterials,8 cancer,9 heart disease,10 and neurodegenerative disease,11 among others.12
When an organic azide is used as the dipole, only certain highly activated nitriles are competent dipolarophiles.13 To-date only a few highly activated nitriles are known to undergo this cycloaddition in an intramolecular fashion with organic azides. When the azide and nitrile moieties are in the same molecule, rate of cycloaddition reaction can be greatly enhanced. Several groups have reported the efficient synthesis of polycyclic fused tetrazoles via intramolecular [3+2] cycloadditin reaction.14
To the best of our knowledge, this report describes the first synthesis of chiral fused tetrazole pyrazine derivatives via an intramolecular [3+2] cycloaddition reaction starting from amino acid derivatives.
In this communication, we report an effective integration of Huisgen’s 1,3 dipolar cycloaddition reaction (one of the prototype reaction in click chemistry) onto natural α-amino acid derivatives for the synthesis of tetrazole-fused pyrazines.
We first devoted our initial efforts toward the synthesis of the key intermediate 12a from L-phenylalanine (6). Thus, Boc protected L-phenylalaninol (7) was prepared from L-phenylalanine (6) by reduction with I2 and NaBH4 in THF followed by Boc-protection with Boc2O and TEA in CH2Cl2 according to the procedure reported in literature.15 Tosyl protection of 7 was carried out by treatment with p-TsCl in pyridine at ambient temperature in good yield. Tosylate 8 was converted to azido derivative 9 by SN2 displacement with NaN3 in DMF at 70 oC in 94% yield. A characteristic peak at 2098 cm-1 in the IR spectrum indicated the presence of the azide functional group. However, the next step, which was to introduce nitrile functionality in azide 9 using bromoacetonitrile was unsuccessful under different reaction conditions (Scheme 1).
To overcome this failure, we at first deprotected Boc with 4N HCl-EtOAc at 0 oC and then nitrile functionality was introduced by treatment with K2CO3 and bromoacetonitrile in CH3CN at room temperature to obtain 11. The structure of 11 was established by NMR spectroscopy and mass spectrometry studies. The secondary amine group of compound 11 was protected with benzyl bromide and K2CO3 at 80 oC to afford 12a in 94% yield. 1H NMR, 13C NMR, mass profile and elemental analysis confirmed the structure of 12a. In the IR spectrum, a strong peak appearing at 2102 cm-1 indicated the presence of both azide and nitrile group. The structure was also confirmed by mass spectrum (ESI-MS), ion peak at m/z = 306 attributed to [M+H]+. As per our previously reported conditions for 1,3-dipolar cycloaddition reaction,16 heating the azido-nitrile derivative 12a in CHCl3 or CH2Cl2 resulted no formation of the cyclic product, the starting material remain unchanged. Then azido-nitrile 12a was heated at 140 oC in DMF for 8 h under reagent free condition to convert tetrazole-fused pyrazine 13a.
Simple purification by silica gel column chromatography afforded the product 13a with excellent yield (90%). The structure of bicyclic tetrazole 13a was established by NMR spectroscopy, mass spectroscopy and elemental analysis. The characteristic resonances observed at δ 149.7 and 56.9 ppm, respectively, were attributed to quarternary and methylene carbon adjacent to double bond. Resonances due to the rest of the carbons were appeared at their expected region. The product was also confirmed by the presence of peaks at m/z = 306 and 328 attributed to [M+H]+ and [M+Na]+ in its ESI-MS spectra.17 Finally, single crystal X-ray crystallography analysis unambiguously confirmed the assigned structure (Figure 2).18-20 It is noteworthy to mention here that 13a was also obtained with almost similar yield when compound 11 was treated with K2CO3, benzyl bromide in DMF at 140 oC.
These results encouraged us to verify the feasibility of this cycloaddition reaction using the other benzyl protected azido-nitriles obtained from different natural amino acids under identical reaction conditions. As examplified in Table 1, the reaction proceeded smoothly to completion, and the corresponding tetrazole-fused 4,5,6,7-tetrahydropyrazine products were obtained in 8 to 12 h with excellent yield and high purity. All bicyclic tetrazole-fused products were fully characterized by NMR spectroscopy, mass spectra and elemental analysis.21
We then decided to extend this reaction condition to L-proline (14) in order to obtain tetrazole-fused tricyclic compound 18. The azido-nitrile 17 obtained from L-proline (14) was heated in DMF at 140 oC to afford the corresponding tetrazole-fused 4,5,6,7-tetrahydropyrazine (18) in 88% yield (Scheme 3). The spectroscopic and analytical data established the structure.21
In conclusion, we have designed and synthesized a series of new chiral fused bicyclic and tricyclic tetrazole derivatives of biologically important starting from easily available α-amino acids and its derivatives. Structural resemblance of these new compounds with substances possessing important pharmacological properties suggests interesting bioactivity. This study is currently being performed on our synthesized compounds and the results will be presented in due course.
ACKNOWLEDGEMENT
PKM and RVG thank CSIR, New Delhi and NCL, Pune, for the financial assistance in the form of research fellowship. We are thankful to Dr. Ganesh Pandey, HOD, Organic Chemistry Division, for his constant support and encouragement. We thank also Dr. Mohan M. Bhadbhade, Dr. Rajesh G. Gonnade and Dr. P. R. Rajmohanan for the X-ray crystallographic assistance and NMR data, respectively.
This paper is dedicated to Professor Emeritus Keiichiro Fukumoto on the occasion of his 75th birthday.
References
1. (a) D. A. Horton, G. T. Bourne, and M. L. Smythe, Chem. Rev., 2003, 103, 893; CrossRef (b) M. R. Grimmett, Compr. Heterocycl. Chem. II., 1996, 3, 77.
2. (a) J. Zabrocki, G. D. Smith, Jr. J. B. Dunbar, H. Iijima, and G. R. Marshall, J. Am. Chem. Soc., 1988, 110, 5875; CrossRef (b) P. A. Bartlett and F. Acher, Bull. Soc. Chim. Fr., 1986, 771; (c) J. S. Morley, J. Chem. Soc., 1969, 809; (d) H. Singh, A. S. Chawla, V. K. Kapoor, D. Paul, and R. K. Malhotra, Prog. Med. Chem., 1980, 17, 151. CrossRef
3. D. Moderhack, J. Pract. Chem., 1998, 340, 687. CrossRef
4. (a) F. Ek, L.-G. Wistrand, and T. Frejd, Tetrahedron, 2003, 59, 6759; CrossRef (b) V. Aureggi and G. Sedelmeier, Angew. Chem.Int. Ed., 2007, 119, 8592. CrossRef
5. S. Daya, P. T. Kaye, and M. Mphahlele, J. Med. Sci. Res., 1996, 24, 137.
6. M. E. Jung, H. Lal, and M. B. Gatch, Neurosci. Biobehavioral, Rev., 2002, 26, 429. CrossRef
7. (a) B. Devis, T. W. Brandstetter, C. Smith, L. Hackett, B. G. Winchester, and G. W. J. Fleet, Tetrahedron Lett., 1995, 36, 7507; CrossRef (b) T. W. Brandstetter, B. Devis, D. Hyett, C. Smith, L. Hackett, B. G. Winchester, and G. W. J. Fleet, Tetrahedron Lett., 1995, 36, 7511. CrossRef
8. (a) E. E. Smissman, A. Terada, and S. Ei-Antably, J. Med. Chem., 1976, 19, 165; CrossRef (b) A. Fleming, Brit J Exper Path., 1929, 10, 226; (c) H. Florey, Conquest, 1953, 41, 4.
9. (a) J. J. McGuire, C. A. Russell, W. E. Bolanowska, C. M. Freitag, C. S. Jones, and T. I. Kalman, Cancer Res., 1990, 50, 1726; (b) F. Itoh, K. Yukishige, M. Wajima, K. Ootsu, and H. Akimoto, Chem. Pharm. Bull., 1995, 43, 230; (c) A. Sobrero and J. R. Berlino, Clinical aspects of drug resistance. Cancer Surv., 1986, 5, 93.
10. J. A. Zablocky, M. Miyano, N. R. Sashidhar, S. Panzer-Knodle, N. Nicholson, and L. Feigen, J. Med. Chem., 1992, 35, 4914. CrossRef
11. (a) W. H. Lunn, D. D. Schoepp, D. O. Calligaro, R. T. Vasileff, L. J. Heinz, C. R. Salhoff, and P. J. O’Malley, J. Med. Chem., 1992, 35, 4608; CrossRef (b) P. L. Ornstein, M. B. Arnold, D. Evrard, J. D. Leander, D. Lodge, and D. D. Shoepp, Bioorg. Med. Chem. Lett., 1993, 3, 43; CrossRef (c) E. H. F. Wong, J. Kemp, and A. Annu, Rev. Pharmacol. Toxicol., 1991, 31, 491.
12. Z. P. Demko, and K. B. Sharpless, Org. Lett., 2002, 4, 2525. CrossRef
13. (a) H. Quest and L. Bieber, Tetrahedron Lett., 1976, 18, 1485; CrossRef (b) I. V. Zavarzin, V. M. Zhulin, V. N. Yarovenko, and M. M. Krayushkin, Izv. Akad. Nauk SSSR, Ser. Khim., 1988, 5, 1168; (c) D. H. Klaubert, J. H. Sellstedt, C. J. Guinosso, S. C. Bell, and R. J. Capetola, J. Med. Chem., 1981, 24, 748; CrossRef (d) Z. R. Demko and K. B. Sharpless, Angew. Chem., Int. Ed., 2002, 12, 2110; CrossRef (e) Z. R. Demko and K. B. Sharpless, Angew. Chem. Int. Ed., 2002, 12, 2113. CrossRef
14. (a) Z. P. Demko and K. B. Sharpless, Org. Lett., 2001, 3, 4091; CrossRef (b) M. S. Taylor, D. N. Zalatan, A. M. Lerchner, and E. N. Jacobsen, J. Am. Chem. Soc., 2005, 127, 1313. CrossRef
15. M. J. McKennon and A. I. Meyers, J. Org. Chem, 1993, 58, 3568. CrossRef
16. (a) D. K. Mohapatra, P. K. Maity, R. G. Gonnade, M. S. Chorghade, and M. K. Gurjar, Synlett, 2007, 1893; (b) D. K. Mohapatra, P. K. Maity, M. S. Chorghade, and M. K. Gurjar, Heterocycles, 2007, 73, 269. CrossRef
17. Compound 12a: mp 102 °C; [α]25D -16.4 (c 1.1, CHCl3); IR (CHCl3): 3369, 3027, 2928, 2102, 1601, 1454, 1273, 1125 cm-1; 1H NMR (CDCl3, 400 MHz): δ = 2.80 (dd, 1H, J = 9.3, 13.1 Hz), 3.19 (dd, 1H, J = 5.3, 13.1 Hz), 3.25 (m, 1H), 3.46 (s, 2H), 3.60 (s, 2H), 3.99 (s, 2H), 7.20 (d, 2H, J = 7.3 Hz), 7.34 (m, 8H); 13C NMR (CDCl3, 100 MHz): δ = 35.0, 38.3, 51.0, 54.6, 63.8, 116.7, 126.7, 127.9, 128.8, 129.1, 136.8, 138.1; ESI-MS: m/z = 306 [M+H]+. Anal. Calcd (%) for C18H19N5: C, 70.80; H, 6.27; N, 22.93. Found: C, 70.95; H, 6.12; N, 23.18. Compound 13a: mp = 106-108 °C; [α]25D -6.3 (c 1.6, CHCl3); IR (CHCl3): 3401, 2924, 1601, 1454, 1118 cm-1; 1H NMR (CDCl3, 400 MHz): δ = 2.52 (dd, 1H, J = 10.5, 13.4 Hz), 3.16 (dd, 1H, J = 4.6, 13.4 Hz), 3.55 (m, 1H), 3.87 (d, 1H, J = 13.0 Hz), 3.98 (d, 1H, J = 13.0 Hz), 4.10 (d, 1H, J = 16.0 Hz), 4.18-4.23 (m, 2H), 4.34 (dd, 1H, J = 3.3, 13.0 Hz), 7.09 (d, 2H, J = 7.3 Hz), 7.29-7.40 (m, 8H); 13C NMR (CDCl3, 100 MHz): δ = 31.7, 43.6, 46.5, 56.7, 56.9, 126.9, 127.8, 128.5, 128.6, 128.8, 128.9, 136.7, 136.9, 149.7; ESI-MS m/z = 306 [M+H]+, 328 [M+Na]+. Anal. Calcd (%) for C18H19N5: C, 70.80; H, 6.27; N, 22.93. Found: C, 71.03; H, 6.40; N, 22.67.
18. X-Ray intensity data was collected on Bruker SMART APEX CCD diffractometer with graphite-monochromatized (MoKα = 0.71073 Ǻ) radiation at room temperature. All the data were corrected for Lorentzian, polarization and absorption effects using Bruker’s SAINT and SADABS programs. SHELX-97 (ShelxTL)22 was used for structure solution and full matrix least squares refinement on F2. Hydrogen atoms were included in the refinement as per the riding model.
19. Sheldrick, G. M. SHELX-97 Program for Crystal Structure Solution and Refinement; University of Gottingen: Germany, 1997.
20. Crystallographic data for the structures in this paper have been deposited with the Cambridge Crystallographic Data Centre as supplementary publication number CCDC 705302 for 13a. Copies of the data can be obtained, free of charge, on application to CCDC, 12 Union Road, Cambridge, CB2 1EZ, UK [fax: +44 (1223) 336033; or e-mail: deposit@ccdc.cam.ac.uk].
21. Compound 13b: [α]25D +4.6 (c 1.2, CHCl3); IR (CHCl3): 3369, 2972, 1600, 1448, 1219 cm-1; 1H NMR (CDCl3, 400 MHz): δ = 1.24 (d, 3H, J = 6.7 Hz), 3.37-3.45 (m, 1H), 3.69 (d, 1H, J = 13.0 Hz), 3.86 (d, 1H, J = 13.0 Hz), 3.95 (ABq, 2H, J = 16.7 Hz), 4.19 (dd, 1H, J = 5.0, 12.6 Hz), 4.44 (dd, 1H, J = 4.6, 12.6 Hz), 7.32 (m, 5H); 13C NMR (CDCl3, 100 MHz): δ = 12.4, 43.9, 50.3, 51.0, 56.8, 127.8, 128.7, 136.8, 149.8; ESI-MS m/z = 230 [M+H]+, 252 [M+Na]+. Anal. Calcd (%) for C12H15N5: C, 62.86; H, 6.59; N, 30.54. Found: C, 62.91; H, 6.42; N, 30.78. Compound 13e: [α]25D -6.0 (c 1.1, CHCl3); IR (CHCl3): 3402, 2957, 1600, 1453, 1367, 1219, 1074 cm-1; 1H NMR (CDCl3, 400 MHz): δ = 0.95 (t, 6H, J = 7.0 Hz), 1.21-1.29 (m, 1H), 1.57-1.64 (m, 1H), 1.73-1.83 (m, 1H), 3.37 (m, 1H), 3.60 (d, 1H, J = 13.2 Hz), 3.82 (d, 1H, J = 13.2 Hz), 4.05 (ABq, 2H, J = 17.2 Hz), 4.26 (dd, 1H, J = 4.8, 12.9 Hz), 4.44 (dd, 1H, J = 4.8, 12.9 Hz), 7.28-7.36 (m, 5H); 13C NMR (CDCl3, 100 MHz): δ = 22.5, 22.7, 24.9, 36.3, 43.5, 46.9, 53.4, 55.3, 127.9, 128.6, 128.7, 137.0, 149.6; ESI-MS m/z = 272 [M+H]+, 294 [M+Na]+. Anal. Calcd (%) for C15H21N5: C, 66.39; H, 7.80; N, 25.81. Found: C, 66.18; H, 7.71; N, 25.96. Compound 17: [α]25D -88.2 (c 1.7, CHCl3); IR (CHCl3): 3391, 2968, 2101, 1646, 1224, 1277, 1044 cm-1; 1H NMR (CDCl3, 200 MHz): δ = 1.58-1.73 (m, 1H), 1.77-1.91 (m, 2H), 1.93-2.07 (m, 1H), 2.70 (ABq, 1H, J = 8.5 Hz), 2.84-2.96 (m, 1H), 3.01-3.11 (m, 1H), 3.22 (dd, 1H, J = 5.9, 12.5 Hz), 3.39 (dd, 1H, J = 4.6, 12.5 Hz), 3.76 (ABq, 2H, J = 17.6 Hz); 13C NMR (CDCl3, 50 MHz): δ = 22.8, 28.5, 40.8, 53.4, 54.0, 60.2, 115.1; ESI-MS m/z = 166 [M+H]+, 188 [M+Na]+. Anal. Calcd (%) for C7H11N5: C, 50.89; H, 6.71; N, 42.39. Found: C, 51.15; H, 6.49; N, 42.50. Compound 18: [α]25D +60.1 (c 0.6, CHCl3); IR (CHCl3): 2923, 1654, 1384, 1220, 1074 cm-1; 1H NMR (CDCl3, 400 MHz): δ = 1.64-1.74 (m, 1H), 1.98-2.08 (m, 2H), 2.12-2.21 (m, 1H), 2.44 (q, 1H, J = 8.8 Hz), 2.73-2.81 (m, 1H), 3.29-3.34 (m, 1H), 3.56 (d, 1H, J = 15.7 Hz), 3.99 (t, 1H, J = 11.4 Hz), 4.52 (d, 1H, J = 15.5 Hz), 4.68 (dd, 1H, J = 3.9, 12.2 Hz); 13C NMR (CDCl3, 100 MHz): δ = 22.8, 27.5, 47.3, 50.8, 53.5, 59.5, 151.2; ESI-MS m/z = 166 [M+H]+, 188 [M+Na]+. Anal. Calcd (%) for C7H11N5: C, 50.89; H, 6.71; N, 42.39. Found: C, 50.74; H, 6.97; N, 42.42.