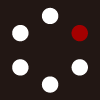
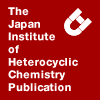
HETEROCYCLES
An International Journal for Reviews and Communications in Heterocyclic ChemistryWeb Edition ISSN: 1881-0942
Published online by The Japan Institute of Heterocyclic Chemistry
e-Journal
Full Text HTML
Received, 6th October, 2009, Accepted, 20th November, 2009, Published online, 20th November, 2009.
DOI: 10.3987/REV-09-661
■ Total Synthesis of Strychnine
Miwako Mori*
Faculty of Pharmaceutical Sciences, Health Sciences University of Hokkaido, Ishikari-Tobetsu, Hokkaido 061-0293, Japan
Abstract
Strychnine is one of the most famous and complex natural products in its size. Woodward succeeded in the total synthesis of (-)-strychnine in 1954, but there were no other reports of the total synthesis of strychnine for about 40 years. In 1992, Magnus succeeded in the total synthesis of (-)-strychnine and then due to the dramatic progress of synthetic organic chemistry and organometallic chemistry, many researchers tried to synthesize (±)- and (-)-strychnine by their original methods. We developed a novel synthetic method of a chiral cyclohexenyl amine derivative having an aryl group at the 2-posotion by palladium catalyst for the synthesis of (-)-mesembrine. Furthemore, (+)-crinamine, (-)-haemanthidine, and (+)-pretazettine could be synthesized by the same procedure. Modifying this method, the total synthesis of (-)-strychnine was achieved. The chiral cyclohexenyl amine derivative having the sylyloxymethyl group at the 2-position was synthesized using the palladium catalyst. From this comopound, construction of the ABE-rings of (-)-strychnine was carried out. The C-ring was constructed using palladium-catalyzed allylic oxidation. The G-ring and then the F-ring were constructed by intramolecular Heck reaction. All cyclizations for synthesis of (+)-isostrychnine were performed using palladium catalysts. (+)-Isostrychnine was converted into (-)-strychnine by the known method. The progress of the synthesis of a chiral cyclohexenyl amine derivative having the substituent at the 2-position was described.CONTENTS
1. Introduction
2. Earlier Total Syntheses of Strychnine (~2000)
3. Recent Total Syntheses of Strychnine (2000~)
4. Total Synthesis of (-)-Strychnine by Our Group
4.1. Background on the synthesis of (-)-strychnine
4-1-1. Development of Novel Synthetic Procedure of Chiral Cyclohexenylamine Derivative Having the Substituent at 2-Position
4-1-2.Asymmetric Total Syntheses of (+)-Crinamine, (-)-Haemanthidine, and (+)-Pretazettine
4-1-3.Reaction Mechanism for Asymmetric Allylic Substitution
4-2. Total Synthesis of (-)-Tubifoline
4-3. Total Synthesis of (-)-Strychnine
1. INTRODUCTION
(-)-Strychnine, which was first isolated in 1818 from the seeds and bark of Strychnos nux vomica by Pelletier and Caventous,1 is one of the most famous and complex natural products in its size. It has seven rings and six asymmetric centers in the molecule. Woodward succeeded in the total synthesis of (-)-strychnine in 1954,2 but there were no other reports on the total synthesis of strychnine for about 40 years. However, recently, due to the dramatic progress of synthetic organic chemistry and organometallic chemistry, the total synthesis of strychnine was achieved by several groups.3 The numbering system and ring laveling used throughout this review is shown in Figure 1.4
In 1992, Magnus succeeded in the total synthesis using a relay compound obtained from (-)-strychnine.5 Overman prepared the starting material by an enzymatic desymmetrization and succeeded in the first asymmetric total synthesis of (-)- and (+)-strychnine in 1993.6 Kuehne7 succeeded in the total synthesis of (-)-strychnine from a chiral pool, L-tryptophane, and Bosch7 used diastereoselective reductive double amination for the synthesis of perhydroindolone derivative as an intermediate. Following these reports, several groups succeeded in the total synthesis of (-)- or (±)-strychnine.3 Rawal’s synthetic process is particularly remarkable, although it is a racemic form.8 Very recently, Vollhardt succeeded in the total synthesis of (±)-strychnine using an ingenious cobalt-catalyzed [2+2+2]cycloaddition as a key step.9 However, there has been no report on total synthesis of (-)-strychnine from enantiomerically pure compound obtained by a transition metal-catalyzed asymmetric synthesis. In 2002, we have succeeded in the total synthesis of (-)-strychnine.10 For the chiral starting material, 2-substituted cyclohexenylamine derivative prepared by palladium catalyzed asymmetric allylic substitution was used. In 2004, Shibasaki succeeded in the total synthesis of (-)-strychnine from the chiral starting material prepared by his asymmetric Michael reaction.11 In this review, the progress for development of palladium catalyzed asymmetric allylic substitution developed by our group and the total syntheses of some natural products using this method are reported.
2. EARLIER TOTAL SYNTHESES OF STRYCHNINE (~2000)
An exquisite review of the total synthesis of strychnine was reported by Bonjoch and Sole in 2000.3c Thus, in this chapter, only the first total synthesis of (-)-strychnine reported by Woodward2 and Rawal’s total synthesis8 of (±)-strychnine are shown. The first total synthesis of (-)-strychnine by Woodward in 1954 is a memorial affair in the field of synthetic organic chemistry. In the Rawal’s total synthesis of strychnine, which was achieved by two researchers, we can find the progression of synthetic organic chemistry using the transition metal complex.
The brilliant total synthesis of strychnine by Woodward is shown in Schemes 1 and 2. He started from an indole derivative (1), prepared by Fischer indole synthesis, which was converted into a tryptamine derivative (2). Condensation of 2 with ethyl glyoxylate followed by treatment with TsCl gave the spiro compound (3), which was treated with NaBH4 followed by acetylation to give the compound (4). The next pyridone formation is the most impressive process for the synthesis of strychnine. When the compound (4) was treated with ozone in aqueous acetic acid, the veratryl group was selectively cleaved between the two methoxy groups to give the muconic ester (5), which was heated in methanolic hydrogen chloride to afford the pyridone (6). To construct of E-ring, Dieckman condensation was carried out to give 8, whose keto-carbonyl group was removed via the thienol ether (9) and then hydrolysis of the ester group afforded 10 with the more stable equatorial orientation for the carboxyl group (Scheme 1).
Acetylation of 10 gave 13. This reaction involves the initial formation of a mixed anhydride (11), which undergoes conversion to a methyl ketone under the reaction condition by evolution of carbon dioxide followed by acetylation. Treatment of 13 with aqueous hydrochloric acid in acetic acid gave the methyl ketone (14), with the more stable equatrial orientation for the acetyl group. Oxidation of 14 with SeO2 in ethanol gave 16 via the keto-aldehyde (15). Reaction of 16 with sodium acetylide followed by hydrogenation with Lindlar catalyst gave an allylic alcohol (17). Treatment of 17 with LiAlH4 gave amine (18), whose pyridone ring was reduced to the desired dihydro level. Acid treatment of 18 gave isostrychnine. Finally, isostrychnine was treated with potassium hydroxide in ethanol to give strychnine (Scheme 2).
Rawal’s total synthesis is notable for the conciseness, short steps (15 steps) and high overall yield (10%).8 They prepared ABCE-rings by intramolecular Diels-Alder reaction. The most unique point of their synthesis is the use of the palladium catalyst for the construction of the D-ring and this procedure affected the later total synthesis of strychnine. They prepared a pyrroline (20) from 2-nitrobenzylcyanide as shown in Scheme 3.
Condensation of 21 with 22 followed by treatment with methyl chloroformate afforded a triene (23). Intramolecular Diels-Alder reaction of 23 smoothly proceeded to give the tetracyclic compound 24 in high yield. Deprotection of the methoxycarbonyl group on nitrogen gave the compound (25), whose amino group was alkylated with 26a to give 27. To construct D-ring, intramolecular Heck reaction12 of 27 was carried out in the presence of the palladium catalyst and the hexacyclic compound (28) was obtained in good yield. Acid treatment of 28 gave isostrychnine. Thus, the total synthesis of (±)-strychnine was achieved (Scheme 4).
3. Recent Total Synthesis of Strychnine (2000~)
After 2000, six groups succeeded in the total synthesis of strychnine. Vollhardt succeeded in the total synthesis of (±)-strychnine using cobart-mediated [2+2+2]cycloaddition reaction in 2000.9 Then our group,10 Bodwell,13 Shibasaki,11 Fukuyama,14 and Padwa15 reported the total synthesis of strychnine. All approaches aimed at the synthesis of isostrychnine or the Wieland-Gumlich aldehyde as the final target molecule because they have already led to strychnine.16
In 2000, Vollhardt reported a short, a highly convergent total synthesis of strychnine using cobalt-mediated [2+2+2]cycloaddition.9 He had already reported the cycloaddition of three unsaturated functionalities using cobalt complex. The reaction proceeded with a high degree of chemo-, regio-, and stereoselectivity and a number of aromatic heterocycles is included as the unsaturated functionalily. The indole nucleus is also active in these cyclizations to provide a polycyclic ring system. For example, tetracyclic compound (29) was synthesized by [2+2+2]cycloaddition of N-acetyl indole and octa-1,7diyne (Scheme 6).
His retrosynthetic analysis of strychnine is shown in Scheme 7. The feature of his synthesis is that the E and G rings are constructed from an indole derivative (31) and an alkyne (32) by his original method.
[2+2+2]Cycloaddition reaction of 35, which was prepared from N-acetyl tryptamine (33) and an ene-yne (34), with acetylene using CpCo(C2H4)2 at 0 °C smoothly proceeded to give the tetracyclic compound (36) in 47% yield. Pyrrolidine ring closure occurred by deacetylation followed by demetalation with iron (III) to give the pentacycle (38) in high yield. To construct D-ring, they examined Rawal’s procedure8 and radical cyclization. Alkylation of amine nitrogen of 38 with 26a followed by base-catalyzed isomerization of the diene unit into conjugation with the amide carbonyl afforded the vinyl iodide (39). Palladium catalyzed Heck reaction of 39 in the presence of a catalytic amount of Pd(OAc)2 and PPh3 gave the hexacyclic compound (40). On the other hand, reaction of compound (39) with Bu3SnH and AIBN afforded the desired hexacyclic silyl ethers (28) and (Z-28) in 71% yield as a 1 : 1 mixture. Treatment of 40 with LiAlH4 gave the same compound (28). Deprotection of the silyl group of 28 followed by treatment with KOH gave (±)-strychnine.
Bodwell reported a concise formal total synthesis of (±)-strychnine using a transannular inverse electron demand Diels-Alder reaction of a [3](1,3)indolo[3](3,6)pyridazinophane.13 The reaction of tryptamine and 3,6-diiodopyridazine gave 42. N-Allylation of the indole moiety yielded 43, which was subjected to a sequential hydroboration/intramolecular B-alkyl Suzuki-Miyaura cross coupling reaction to give cyclophane. Protection of the secondary amine afforded 44, which was heated in N,N-dimethylaniline to induce the pentacyclic compound (46) by expulsion of N2 from 45 in quantitative yield. Reduction of (±)-46 with NaBH4/CF3CO2H occurred with complete chemo- and stereoselectivity to afford 47. Oxidation of the tertiary amine with PDC followed by deprotection gave an amide (25), which is an intermediate for the synthesis of strychnine by Rawal.8 Thus the formal total synthesis of (±)-strychnine was established (Scheme 9).
Shibasaki et al.,11 succeeded in the synthesis of (-)-strychnine from chiral 51 prepared by their highly practical asymmetric Michael reaction. Their retrosynthetic analysis is shown in Scheme 10. To utilize 51 effectively in the synthesis, cyclohexene ring of 51 is used as E-ring and construction of B- and D-rings is carried out from 51. The spirocenter C7 is constructed in the last stage by intramolecular alkylation (Scheme 10).
Only 0.1 mol% of (R)-AlLibis-(binaphtoxide) (ALB) completed the Michael reaction17 to give 51 (> 99% ee). Then 51 was converted into 56 via several steps as shown in Scheme 11. Palladium catalyzed coupling reaction of 56 with ortho-stannylated nitrobenzene (57) smoothly proceeded, and then protection of the hydroxyl group and deprotection of the silyl group gave the key intermediate (50).
After introduction of the amine moiety, the crude product was treated with Zn in MeOH-aqueous NH4Cl to produce 49 via 59. For the construction of C-ring, 49 was treated with DMTSF to afford 60, which was converted into 61. Desulfurization followed by oxidation with SO3-pyridine and then deprotection of the silyl group gave Wieland-Gumlich aldehyde, which was converted into (-)-strychnine (Scheme 12).
Fukuyama succeeded in total synthesis of (-)-strychnine in 2004.14 His retrosynthetic analysis is shown in Scheme 13. Construction of the nine-membered ring is performed by his 2-nitrobenzenesulfonamide (NsNH2) strategy.18 To secure the geometry of the trisubstituted olefin, they planned to cleave the cyclohexene ring at the C3-18 bond of 64 to generate the two side chains. The precursor (64) should be obtained by palladium-catalyzed coupling reaction of 65 and 66.
The starting cyclohexadiene (67) was converted into 68, which was subjected to an enzymatic resolution with lipase AYS to provide the desired bromohydrin acetate (69) with high ee. From 69, the desired epoxide (66) was obtained (Scheme 14).
The palladium-catalyzed coupling reaction of 65 and 66 was carried out in the presence of Pd2(dba)3 and P(2-furyl)3 in toluene to afford 70 in high yield with complete control of regio- and stereochemistry. Then coupling product (70) was converted into the diol (71), which was treated with NsNH2 via double N-alkylation to form the nine-membered ring compound (72). Epimerization of C16, and then deprotection followed by oxidation gave 73, which was converted into 74. Oxidative cleavage of the α-hydroxy ketone (74) with Pb(OAc)4 was performed to afford an aldehyde (75). Removal of the nosyl group followed by treatment with TFA and Me2S induced a transannular cyclization to give the pentacyclic compound (77), which is an intermediate of the Kuehne’s total synthesis of strychnine.7a Thus, 77 was converted into (-)-strychnine via the Wieland-Gumlich aldehyde 13 (Scheme 15).
In 2007, Padwa reported the total synthesis of (±)-strychnine.15 Their retrosynthetic analysis is shown in Scheme 16. The feature of his synthesis is an intramolecular [4 +2] cycloaddition/
rearrangement cascade of an indolyl-substituted amidofuran (81) for the construction of the ABCE rings. Furthermore, they used intramolecular palladium catalyzed coupling reaction of vinyl iodide and α-position of the carbonyl group of 79.
Heating the compound (81), prepared from 82 and 83, at 150 °C (toluene) in a microwave reactor for 30 min in the presence of catalytic amount of MgI2 afforded the desired aza-tetracycle (86) via 84 and then 85 in 95% yield, which was converted into 88. Alkylation of 88 with 26b followed by protection of the amino group and then oxidation afforded the desired compound (79). For the construction of D-ring, palladium catalyzed cyclization was carried out to give the pentacyclic compound (90), which was converted into an enol ether (78). Acid hydrolysis of 78 gave Wieland-Gumlich aldehyde. From this compound total synthesis of (±)-strychnine was achieved (Scheme 17).
4. TOTAL SYNTHESIS OF (-)-STRYCHNINE BY OUR GROUP
4-1. BACKGROUND ON THE SYNTHESIS OF (-)-STRYCHNINE
Zirconium-mediated cyclization is useful method for the synthesis of fused cyclic compounds. We developed this reaction for the synthesis of fused heterocyclic compounds.19 The cyclohexene derivative (91), prepared from (-)-carvone, was treated with Cp2ZrBu2 to give a zirconacycle (92), whose solution was stirred under an atmosphere of carbon monoxide to give 93. From this compound (93), (-)-dendrobine could be synthesized (Scheme 18).19
When the cyclohexenylamine derivative (94) having an aryl group at the 2-position was treated with Cp2ZrBu2 and a solution of 95 was stirred under O2, the indoline derivative (96) having the aryl group at the 8-poistion was synthesized, and the total syntheses of mesembrane and mesembrine were achieved from this compound. As the next problem, how to obtain a chiral 94 for the synthesis of (-)-mesembrane and (-)-mesembrine was considered. Thus, a novel synthesis of a chiral cyclohexenylamine derivative (94) having the aryl group at the 2-position was planed (Scheme 19).
here are many alkaloids that have an aromatic ring connected to a cyclohexane ring. Even in the case of indole alkaloids, these ring systems are found in the molecule. Thus, various indole alkaloids could be synthesized from a chiral cyclohexenylamine derivative having substituent at the 2-position. In this chapter, the progress of the syntheses of chiral indole alkaloids was described.
4-1-1. DEVELOPMENT OF NOVEL SYNTHETIC PROCEDURE OF CHIRAL CYCLOHEXENYLAMINE DERIVATIVE HAVING THE SUBSTITUENT AT 2-POSITION
Palladium-catalyzed alkylation or amination of the compound (97) should give compound (99) via a π-allylpalladium complex (98). If a chiral ligand is used for this reaction, an optically active 99 or ent-99 should be formed from the racemic 97 (Scheme 20).
When a DMSO solution of an allyl carbonate (97a) and an N-tosylallylamine was warmed in the presence of Pd2(dba)3·CHCl3 (2.8 mol%) and dppb (5.6 mol%) as a ligand at 50 °C for 13 h, the desired tosylamide (100) was obtained in 36% yield (Table 1, run 1). The same reaction was carried out in the presence of (S)-BINAP20 or (+)-10121 as a chiral ligand, but the reaction rate was lower and good results were not obtained (runs 2 and 3). However, when the reaction was carried out in the presence of palladium catalyst and (S)-BINAPO,22 the reaction proceeded smoothly at room temperature for 3 h to produce 100 in 51% yield with 70% ee (run 4). With regard to the solvent, non-polar solvent gave good results (runs 4-9). When the reaction was carried out in THF at room temperature for 19 h, the chiral diene (100) was produced in 80% yield with 86% ee (run 9), which was recrystallized from MeOH to give 100 with 99% ee (79% recovery).
Thus, the development of the novel synthetic method of the chiral cyclohexenylamine derivative having the substituent at the 2-position could be achieved. From the compound (100), (-)-mesembrane and (-)-mesembrine were synthesized.23
4.1.2. ASYMMETRIC TOTAL SYNTHESES OF (+)-CRINAMINE, (-)-HAEMANTHIDINE, AND (+)-PRETAZETTINE
Subseqently, the asymmetric total syntheses of the crinane-type alkaloid, (+)-crinamine,24 (-)-haemanthidine, and (+)-pretazettine25 were planned using our method.
If an aldehyde ((S)-103) is obtained from 102 using this asymmetric allylic substitution, the intramolecular carbonyl-ene reaction26 of 103 would construct the quaternary carbon center of 104 in a stereoselective manner. From this compound (104), the target alkaloids would be synthesized in an optically pure form in short steps (Scheme 21).
Initially, the cyclohexenylamine derivative (106) was synthesized from the allyl alcohol (102) and the tosylamide (105). When a THF solution of 102a (1 equiv), 105 (1.1 equiv), Pd2(dba)3·CHCl3 (2.5 mol%), and (S)-BINAPO (5.0 mol%) was stirred at room temperature for 18 h, the desired cyclohexenylamine derivative (106) was obtained in 68% yield with 60% ee as a crystalline product (Table 2, run 1). The ee of 106 was slightly raised when the reaction was carried out at a low temperature, although the reaction time was longer and the yield is moderate (run 2). Then the leaving group was changed to phosphonate. At a low temperature, the desired product (106) was obtained in 80% yield with 74% ee (run 4). As a solvent, THF was the most suitable (runs 4-6), and the use of (S)-BINAP as the ligand gave 106 with a high ee, although the yield was moderate (run 7). When the compound (106) with 74% ee was recrystalyzed from MeOH, the racemic 106 was obtained as colorless crystals, and the concentration of the mother liquor gave the oily (-)-106 with 99% ee.
Deacetalization of 106 (99% ee) with FeCl3•SiO2 gave the aldehyde (103) in high yield. As expected, when a toluene solution of 103 was heated at 230-240 °C in the presence of 4Å molecular sieves for several hours, the desired hexahydroindole (104) was obtained in 58% yield (72% conversion) as a sole product. The NOE experiment with acetylated compound (107) indicates that the ring junction of the 5,6 membered ring is cis and that the acetoxy group is trans to the aryl group. The stereochemistry of this compound is consistent with that of the target alkaloids. Allylic oxidation of 107 with SeO2 gave the alcohol (108) in a highly stereoselective manner as the sole product because SeO2 approached the olefin from the face opposite of the large phenyl group. The reaction of Ms2O with 108 in the presence of NEt3 followed by treatment with MeOH, gave 109a as the main product (β : α, 6.0 : 1). Detosylation of 109a followed by methylenation and deacetylation smoothly proceeded to give (+)-crinamine. Thus, the asymmetric total synthesis of (+)-crinamine was achieved in 9 steps from 102b in 20% overall yield, and this establishes the absolute confuguration of C-3 in (-)-106 to be S (Scheme 22).
Treatment of 108 with HC(OMe)3 in the presence of Montmorillonite K-10 gave 109b in high yield (α : β, 4.0 : 1). Detosylation of 109b followed by formylation, gave the compound (110). Treatment of 110 with POCl3 followed by deacetylation gave (-)-haemanthidine, which could be converted into (+)-pretazettine by a known method. Thus, the total syntheses of (-)-crinamine, (-)-haemanthidine and (+)-pretazettine were achieved (Scheme 23).27
4-1-3. REACTION MECHANISM FOR ASYMMETRIC ALLYLIC SUBSTITUTION
During the course of our study on the total synthesis of (+)-crinamine, (–)-haemanthidine, and (+)-pretazettine, when (±)-97a was reacted with 105 in the presence of Pd2(dba)3·CHCl3 and (S)-BINAPO, the desired product (S)-111 with 83% ee was obtained in 73% yield. We were very surprised to find that the recovered starting material (R)-97a showed 60% ee in 12% yield. This means that kinetic resolution would occur upon the formation of (π-allyl)palladium complex (Scheme 24). The fact that kinetic resolution occurred on palladium-catalyzed enantioselective allylic alkylation was found by Prof. Hayashi, and recently a few group reported in regard to this phenomenon.28
To confirm this, the same reaction was carried out at 0 °C and the time courses of the ees of the product (S)-111 and the starting material (97a) were monitored by HPLC. The results are shown in Table 3. Apparently, kinetic resolution was also shown in this reaction; that is, after 3 h, the ee of the product (S)-111 was 86%, while the ee of the recovered starting material (R)-97a was 30% ee. Although the same ee of (S)-111 was obtained in each time, the ee of the recovered starting material (R)-97a gradually increased, and after 165 h, (R)-97a with 93% ee was obtained in 14% yield along with (S)-111 with 88% ee in 60% yield (Figure 3).
On the other hand, when the reaction of (±)-97a with dimethyl malonate was carried out in the presence of a palladium catalyst and (S)-BINAPO, (–)-112 was obtained in 41% yield but the ee was only 30%. However, the recovered starting material (R)-97a showed 71% ee. When the same reaction was carried out using (±)-97b as the substrate in the presence of NaH, the ee of the recovered starting material was 96%, but the ee of (-)-112 was only 11% (Scheme 25).29
These results indicate that there are two independent pathways in the asymmetric synthesis of (S)-97: that is, kinetic resolution and asymmetric substitution. If the reaction rate of (S)-I with Pd(0) having (S)-BINAPO is faster than that of (R)-I with Pd(0) having (S)-BINAPO, kinetic resolution would occur and (R)-I would remain unchanged. In this process, (R)-I also can react with Pd(0) having (S)-BINAPO to produce the same π-allylpalladium complex. The intermediary (π-allyl)palladium complex II reacts with nucleophile enantioselectively to give (S)-III. Thus, both (S)- and (R)-I can be converted into (S)-III. If the starting material is recovered, (R)-I with a high ee can be obtained (Scheme 26).29
4-2. TOTAL SYNTHESIS OF (-)-TUBIFOLINE
We next turned our attention the total syntheses of strychnine and tubifoline.30 These alkaloids could be synthesized from the tetracyclic ketone (114), which should be an important intermediate for the synthesis of various indole alkaloids as the chiral forms. The compound (114) would be obtained from 115, which would be obtained from 116 by a Heck reaction. If the cyclohexenol derivative (117) having the functional group at the 2-position is reacted with an o-haloaniline derivative (118) in the presence of Pd(0) with a chiral ligand, the cyclohexenyl amine derivative (116) should be obtained as a chiral form (Scheme 27).
To construct an indole skeleton, an o-haloaniline derivatives (118) were used as a nucleophile. When the reaction of 117a and the N-tosylaniline (118b) was carried out in the presence of Pd2(dba)3·CHCl3 and (S)-BINAPO, the cyclohexenylamine derivative (116b) was obtained in 85% yield (Table 4, run 1). The reaction of 117a with the N-tosyl-o-bromoaniline (118a) gave a desired compound (116a) with 80% ee in 78% yield. To enhance the reactivity of the leaving group, a vinyl carbonate (117b) was used, and the reaction was carried out at 0 °C to give 116a with 84% ee in 75% yield (run 3). The lower reaction temperature did not affect the ee of 116a (run 4).
Deprotection of 116a with 4N HCl followed by treatment with PBr3 and then NaCN in DMSO gave a nitrile (119) in good yield. Palladium-catalyzed Heck reaction of 119 proceeded smoothly to give 120 in high yield. The results of an NOE experiment of 120 indicated that the ring junction of the fused 5,6-membered ring is cis. The compound (120) was recrystallized from EtOH to give the optically pure 120 ([α]D -46.7°, 99% ee, 73% recovery). Treatment of 120 with LiAlH4 followed by protection of the primary amine with Boc2O afforded the compound (121). To construct the E-ring, cyclization of 121 was carried out using Pd(II). When an acetic acid solution of 121 was stirred in the presence of 10 mol% of Pd(OAc)2, 40 mol% of benzoquinone and 2 equiv of MnO2 at 50 °C for 15 min, the compound (122) was obtained in 77% yield. Probably, the double bond of 121 coordinates to Pd(II) to form 123 and then amide nitrogen attacks olefin to give the palladium complex (124). Then β-hydrogen elimination from 124 occurs to give 122. Conversion of olefin into ketone was carried out. Based on the results of a modeling study, it was thought that the C-15 position is less hindered than the C-14 position. Thus, it was expected that the hydroxyl group would be introduced at the C-15 position by hydroboration. Hydroboration of 122 using BH3·THF followed by treatment with H2O2 in aq. NaOH gave the alcohols (125) and (125’) in 49% and 51% yields, respectively. The use of a large hydroboration reagent, 9-BBN, afforded the desired alcohols (125) as a major product and an elevated temperature gave the alcohol (125) in 80% yield. Swern oxidation of 125 was carried out to give the desired tetracyclic ketone (126) in high yield. Thus, the synthesis of the tetracyclic ketone (126) from the cyclohexenol derivative (117b) was achieved (Scheme 28).
Subsequently, the synthesis of tusbifoline31 was examined. Treatment of 126 with LDA followed by the addition of PhNTf2 at –78 °C afforded the enol triflates (127) and (128) in 8% and 14% yields, respectively. The base was changed to potassium hexamethyldisilazamide (KHMDS) and the reaction was carried out at –78 °C to give 127 and 128 in 35% yield (ratio of 3 to 2). Since 127 was considered to be the thermodynamic product, the reaction was carried out at –35 °C to afford the desired compound (127) as a sole product in 64% yield. Treatment of the enol triflate (127) with HCO2H in the presence of Pd(OAc)2 and PPh332 gave the desired the olefin (129) in quantitative yield. Deprotection of the tosyl group of 129 with sodium naphthalenide followed by treatment with CF3CO2H gave diamine. Monoalkylation with 130 in the presence of K2CO3 gave 131 in 49% yield from 129. An intramolecular Heck reaction using a palladium catalyst gave a (-)-dehydrotubifoline in 59% yield. Hydrogenation of (-)-dehydrotubifoline with PtO2 in EtOH was carried out to give (-)-tubifoline (Scheme 29).
The results indicated that the absolute configuration of 116a obtained by asymmetric allylic substitution was S. Thus, we succeeded in the total syntheses of (-)-dehydrotubifoline and (-)-tubifolin from the cyclohexenylamine derivative (116a), which was synthesized by palladium-catalyzed asymmetric allylic substitution by 16 steps. All of the steps for the ring constructions were achieved using palladium catalysts.10,33
4-3. TOTAL SYNTHESIS OF (-)-STRYCHNINE
The next target molecule is (-)-strychnine, which should be synthesized from the tetracyclic ketone (126). Our retrosynthetic analysis of (-)-strychnine is shown in Scheme 30. Since the construction of the ABCE-rings of strychnine have been already achieved as a tetracyclic ketone, construction of the G-ring is required for the synthesis of (-)-strychnine. Two pathways should be considered. One is the introduction of an alkyl or an acyl group at the α-position of the carbonyl group of 126 and the formation of a carbon-nitrogen bond for construction of the G-ring. The other is the introduction of an acyl group on nitrogen to form 135 and then construction of the G-ring (Scheme 30).
At first, we chose the former reaction pathway to construct 133 by introduction of an acyl group at the α-position of the carbonyl group in 114. However, many attempts to introduce an acyl group to the α-position of the keto-carbonyl group of 114 were fruitless due to steric hindrance of the large protecting group on aniline nitrogen. Thus, construction of the G-ring by the Heck reaction was tried. Detosylation of 129 followed by treatment with 3-bromoacryloyl chloride in the presence of K2CO3 gave 137, which was treated with 10 mol% of Pd(OAc)2 and 20 mol% of PPh3 in the presence of iPr2NEt in DMSO at 80 °C for 1.5 h to give the pentacyclic compound (138) in 46% yield. Isomerization of the double bond of 138 by NaOiPr in iPrOH followed by deprotection of the Boc group and then alkylation with 26a afforded the compound (39), which is an intermediate for the synthesis of (±)-strychnine reported by Vollhardt (See Scheme 8). Treatment of 39 with Pd(OAc)2, Bu4NCl and K2CO3 in DMF afforded hexacyclic compound (40), which was treated with LiAlH4 followed by deprotection of the silyl group to give (+)-isostrychnine. (+)-Isostrychnine was converted into (-)-strychnine by treatment with KOH in EtOH by the known method (Scheme 31).
Thus, the total synthesis of (-)-strychnine was achieved by 22 steps from 117b.10
In the total synthesis of (-)-strychnine, the starting cyclohexenylamine derivative (116a) was synthesized from 117b by palladium-catalyzed allylic substitution, and all cyclizations for synthesis of (+)-isostrychnine were performed using a palladium catalyst. The compound (116a), obtained from the cyclohexenol derivatives (117b) and (118a) by palladium catalyst, was converted into 121 by palladium catalyzed Heck reaction and it has the ABE-ring system. The C-ring was constructed from the compound (121) using palladium-catalyzed allylic oxidation. The G-ring was formed by palladium-catalyzed cyclization of 137. The D-ring was constructed by Heck reaction of 39. From 39, (-)-strychnine was synthesized. The total synthesis of (-)-strychnine was achieved by 22 steps from 117b (Scheme 32).
The fact that all rings of (-)-tubifoline, (+)-isostrychnine and (-)-strychnine were constructed using a palladium catalyst indicates the importance of a palladium catalyst in modern synthetic organic chemistry.
ACKNOWLEDGEMENT
These works were performed by Dr. S. Kuroda, Dr. C. –S. Zhang, Dr. T. Nishimata, Mr. M. Nakanishi, and Mr. D. Kajishima. I express my heartfelt thanks to them. I also thank to Prof. Y. Sato, Dr. M. Nishida, and Dr. M. Takimoto for their helpful discussion.
References
1. Isolation: P. J. Pelletier and J. B. Caventou, Ann. Chim. Phys., 1818, 8, 323; Structural elucidation: P. J. Pelletier and J. B. Caventou, Ann. Chim. Phys., 1819, 10, 142; L. H. Briggs, H. T. Openshaw, and R. Robinson, J. Chem. Soc., 1946, 903; CrossRef R. B. Woodward and W. J. Brehm, J. Am. Chem. Soc., 1948, 70, 2107. CrossRef
2. R. B. Woodward, M. P. Cava, W. D. Ollis, A. Hunger, H. U. Daeniker, and K. J. Schenker, J. Am. Chem. Soc., 1954, 76, 4749; CrossRef R. B. Woodward, M. P. Cava, W. D. Ollis, A. Hunger, H. U. Daeniker, and K. Schenker, Tetrahedron, 1963, 19, 247. CrossRef
3. For a review of the total synthesis of strychnine; G. F. Smith, In The Alkaloids; ed. by R. H. F. Manske, Academic Press, New York, 1965, p. 591; J. E. Saxton, Alkaloids, Wiley, New York, 1988, Chapters 1 and 7; H. P. Husson, In Indoles, Monoterpene indole alkaloids; ed. by J. E. Saxton, Wiley, New York, 1988, Chapters 1 and 7; J. Bonjoch and D. Solé, Chem. Rev., 2000, 100, 3455; CrossRef M. Shibasaki and T. Ohshima, The Alkaloids, 2007, Vol. 64, 103. CrossRef
4. The numbering system and ring labeling based on the biogenetic interrelationship of indole alkaloids is used. J. Le Men and W. Taylor, Experientia, 1965, 21, 508. CrossRef
5. P. Magnus, M. Giles, R. Bonnert, G. Johnson, L. McQuire, M. Deluca, A. Merritt, C. S. Kim, and N. Vicker, J. Am. Chem. Soc., 1993, 115, 8116. CrossRef
6. S. D. Knight, L. E. Overman, and G. Pairaudeau, J. Am. Chem. Soc., 1993, 115, 9293; CrossRef S. D. Knight, L. E. Overman, and G. Pairaudeau, J. Am. Chem. Soc., 1995, 117, 5776. CrossRef
7. Enantioselective syntheses: M. E. Kuehne and F. Xu, J. Org. Chem., 1998, 63, 9427; CrossRef D. Solé, J. Bonjoch, S. García-Rubio, E. Peidró, and J. Bosch, Angew. Chem. Int. Ed., 1999, 38, 395; CrossRef Racemic syntheses: G. Stork, Reported at the Ischia Advanced School of Organic Chemistry, Ischia Porto, Italy, September 21, 1992; M. E. Kuehne and F. Xu, J. Org. Chem., 1993, 58, 7490. CrossRef
8. V. H. Rawal and S. Iwasa, J. Org. Chem., 1994, 59, 2685. CrossRef
9. M. J. Eichberg, R. L. Dorta, K. Lamottke, and K. P. C. Vollhardt, Org. Lett., 2000, 2, 2479; CrossRef M. J. Eichberg, R. L. Dorta, D. B. Grotjahn, K. Lamottke, M. Schmidt, and K. P. C. Vollhardt, J. Am. Chem. Soc., 2001, 123, 9324. CrossRef
10. M. Nakanishi and M. Mori, Angew. Chem. Int. Ed., 2002, 41, 1934; CrossRef M. Mori, M. Nakanishi, D. Kajishima, and Y. Sato, J. Am. Chem. Soc., 2003, 125, 9801. CrossRef
11. T. Ohshima, Y. Xu. R. Takita, S. Shimizu, D. Zhong, and M. Shibasaki, J. Am. Chem. Soc., 2002, 124, 14546; CrossRef T. Ohshima, Y. Xu, R. Takita, and M. Shibasaki, Tetrahedron, 2004, 60, 9569. CrossRef
12. For a recent review on the Heck reaction, see: R. F. Heck, Vinyl Substitution with Organopalladium Intermediates. In Comprehensive Organic Synthesis, ed. by B. M. Trost, Pergamon Press: New York, 1992, Vol. 4, p. 842.
13. G. J. Bodwell and J. Li, Angew. Chem. Int. Ed., 2002, 41, 3261. CrossRef
14. Y. Kaburagi, H. Tokuyama, and T. Fukuyama, J. Am. Chem. Soc., 2004, 126, 10246. CrossRef
15. H. Zhang, J. Boonsombat, and A. Padwa, Org. Lett., 2007, 9, 279. CrossRef
16. H. Wieland and W. Gumlich, Liebig Ann. Chem., 1932, 494, 191; CrossRef H. Wieland and K. Kaziro, Liebig Ann. Chem., 1933, 506, 60; CrossRef For conversion of isostrychnine to strychnine; V. Prelog, J. Battegay, and W. I. Taylor, Helv. Chim. Acta, 1948, 31, 2244; CrossRef For conversion of Wieland-Gumlich aldehyde into strychnine, see F. A. L. Anet, and R. Lobinson, Chem. Ind., 1953, 245; Formation of isostrychnine from strychnine; H. Leuchs and H. Schulete, Chem. Ber., 1942, 75, 1522. CrossRef
17. M. Shibasaki, H. Sasai, and T. Arai, Angew. Chem. Int. Ed., 1997, 36, 1236; CrossRef S. Shimizu, K. Ohori, T. Arai, H. Sasai, and M. Shibasaki, J. Org. Chem., 1998, 63, 7547; CrossRef Y. Xu, K. Ohori, T. Ohshima, and M. Shibasaki, Tetrahedron, 2002, 58, 2585. CrossRef
18. T. Kan and T. Fukuyama, Chem. Commun., 2004, 353; CrossRef T. Kan and T. Fukuyama, J. Synth. Org. Chem. Jpn., 2001, 59, 779.
19. M. Mori, N. Uesaka, and M. Shibasaki, J. Org. Chem., 1992, 57, 3519; CrossRef M. Mori, F. Saitoh, N. Uesaka, K. Okamura, and T. Date, J. Org. Chem., 1994, 59, 4993; CrossRef N. Uesaka, F. Saitoh, M. Mori, and M. Shibasaki, K. Okamura, and T. Date, J. Org. Chem., 1994, 59, 5633; CrossRef N. Uesaka, M. Mori, K. Okamura, and T. Date, J. Org. Chem., 1994, 59, 4542. CrossRef
20. R. Noyori and T. Takaya, Acc. Chem. Res., 1990, 23, 345. CrossRef
21. B. M. Trost, D. L. Van Vranken, and C. Bingel, J. Am. Chem. Soc., 1992, 114, 9327. CrossRef
22. R. H. Grubbs and R. A. DeVries, Tetrahedron Lett., 1977, 18, 1879; CrossRef B. M. Trost and D. J. Murphy, Organometallics, 1985, 4, 1143. CrossRef
23. M. Mori, S. Kuroda, C.–S. Zhang, and Y. Sato, J. Org. Chem., 1977, 62, 3263. CrossRef
24. Isolations of (+)-crinamine; L. H. Mason, E. R. Puschett, and W. C. Wildman, J. Am. Chem. Soc., 1955, 77, 1253; CrossRef S. Kobayashi, T. Tokumoto, M. Kihara, Y. Imakura, T. Shingu, and Z. Taira, Chem. Pharm. Bull., 1984, 32, 3015; Total synthesis of (±)-crinamine; K. Isobe, J. Taga, and Y. Tsuda, Tetrahedron Lett., 1976, 17, 2331. CrossRef
25. Isolations of 10.1002/cber.19540870922(-)-haemanthidine; H. G. Boit, Chem. Ber., 1954, 87, 1339; CrossRef S. Takagi and M. Yamaki, Yakugaku Zasshi, 1974, 94, 617; Isolation of (+)-pretazettine; W. C. Wildman and D. T. Bailey, J. Org. Chem., 1968, 33, 3749; CrossRef Total syntheses of (±)-haemanthidine and (±)-pretazettine; J. B. Hendrickson, T. L. Bogard, and M. E. Fisch, J. Am. Chem. Soc., 1970, 92, 5538; CrossRef Y. Tsuda, A. Ukai, and K. Isobe, Tetrahedron Lett., 1972, 13, 3153; CrossRef S. F. Martin and S. K. Davidsen, J. Am. Chem. Soc., 1984, 106, 6431; CrossRef Formal total synthesis of (±)-haemanthidine and (±)-pretazettine; H. Ishibashi, H. Nakatani, S. Iwami, T. Sato, N. Nakamura, and M. Ikeda, J. Chem. Soc., Chem. Commun., 1989, 1767. CrossRef
26. Recent review; K. Mikami and M. Shimizu, Chem. Rev., 1992, 92, 1021. CrossRef
27. T. Nishimata and M. Mori, J. Org. Chem., 1998, 63, 7586. CrossRef
28. T. Hayashi, A, Yamamoto, and Y. Ito, J. Chem. Soc., Chem. Commun., 1986, 1090. CrossRef
29. T. Nishimata, K. Yamaguchi, and M. Mori, Tetrahedron Lett., 1999, 40, 5713. CrossRef
30. For the isolation and structural elucidation of this alkaloid, see: W. G. Kump, M. B. Patel, J. M. Rowson, and H. Schmid, Helv. Chim. Acta, 1964, 47, 1497. CrossRef
31. Synthesis of strychnine; B. A. Dadson, J. Harley-Mason, and G. H. Foster, Chem. Commun., 1968, 1233; CrossRef S. Takano, and M. Hirama, and K. Ogasawara, Tetrahedron Lett., 1982, 23, 881; CrossRef K. Shin, M. Moriya, and K. Ogasawara, Tetrahedron Lett., 1998, 39, 3765; CrossRef S. Kogyoku and K. Ogasawara, Heterocycles, 1999, 50, 427; CrossRef Y. Ban, K. Yoshida, J. Goto, T. Oishi, and E. Takeda, Tetrahedron, 1983, 39, 3657; CrossRef M. Amat, A. Linares, and J. Bosch, J. Org. Chem., 1990, 55, 6299; CrossRef M. Amat, M.-D. Coll, J. Bosch, E. Espinosa, and E. Molins, Tetrahedron: Asymmetry, 1997, 8, 935. CrossRef
32. S. Cacchi, E. Morera, and G. Ortar, Tetrahedron Lett., 1984, 25, 4821. CrossRef
33. M. Mori, M. Nakanishi, D. Kajishima, and Y. Sato, Org. Lett., 2001, 3, 1913. CrossRef