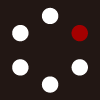
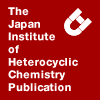
HETEROCYCLES
An International Journal for Reviews and Communications in Heterocyclic ChemistryWeb Edition ISSN: 1881-0942
Published online by The Japan Institute of Heterocyclic Chemistry
e-Journal
Full Text HTML
Received, 30th June, 2014, Accepted, 16th July, 2014, Published online, 22nd July, 2014.
DOI: 10.3987/COM-14-S(K)57
■ Mn(III)-Based Oxidative Cyclization of N-Aryl-3-oxobutanamides. Facile Synthesis and Transformation of Substituted Oxindoles
Nobutaka Kikue, Tetsuya Takahashi, and Hiroshi Nishino*
Department of Chemistry, Graduate School of Science and Technology, Kumamoto University, Kurokami 2-39-1, Chûou-Ku, Kumamoto 860-8555, Japan
Abstract
The oxidation of 3-oxo-N-phenylbutanamides 1 with manganese(III) acetate in ethanol afforded dimeric 3,3'-biindoline-2,2'-dione derivatives 3–5. A similar reaction of N,2-disubstituted N-aryl-3-oxobutanamides 6 in acetic acid produced 3-acetylindolin-2-ones 7 bearing various substituents in good to excellent yields. The acetylindolinones 7 were easily deacetylated by treatment using neutral alumina in diethyl ether. Both the acetylindolinones 7 and deacetylated indolinones 8 were transformed by reduction into the substituted 1H-indoles.INTRODUCTION
Many of the chemistries for indoles and their derivatives have been investigated and reported.1 However, the synthesis and reaction of these heterocycles are still attractive from the view point of the synthetic method,2 total synthesis of natural products,3 biological and pharmacological activities,1b,4 and material science.5 Recently, we reported the Mn(III)-mediated direct substitution of methoxynaphthalenes with N-aryl-3-oxobutanamides, giving the 3-oxobutanamide-substituted naphthalene I in addition to a small amount of demethoxylated naphthofuran II and benzoindolinone III (Scheme 1, eq. 1).6 Although the yield of the heterocyclic compounds II and III was poor, the carbon-carbon bond formation efficiently occurred during the reaction.7 We also reported the facile synthesis of 3,4-dihydro-2(1H)-quinolinones, such as IV, by the Mn(III)-based oxidative cyclization of 2-(2-arylamino-2-oxoethyl)malonates (Scheme 1, eq. 2).8 In the reaction, the formal 6-endo-trig-type cyclization (cyclization at the ortho position) was superior to the formal 5-exo-trig-type (ipso-cyclization) except for the case of the malonates bearing an electron-rich aryl group, producing spiro compounds, such as V. It is also known that 2’-hydroxychalcone undergoes the 5-exo-trig cyclization to afford the aurone.9 In either event, it shows that the construction of heterocycles using the Mn(III)-based carbon-carbon bond formation is convenient.10 In order to favor the 5-exo-trig cyclization using the reaction, N-aryl-3-oxobutanamides 1 should be the most appropriate candidate for the synthesis of nitrogen heterocycles, such as oxindoles (indolin-2-ones). It prompted us to investigate this reaction. In this paper, we describe the facile synthesis of substituted indolin-2-ones using the Mn(III)-based oxidation of N-aryl-3-oxobutanamides and transformation into the corresponding 1H-indoles.
RESULTS AND DISCUSSION
We first prepared the 3-oxo-N-phenylbutanamides 1a-c from the reaction of the corresponding anilines with diketene, and examined the reaction with manganese(III) acetate, Mn(OAc)3 (Scheme 2). The reaction was carried out in acetic acid at reflux temperature similar to the oxidation of malonates as shown in Scheme 1, eq. 2.8 Although the oxidation finished within 2-3 minutes along with some recovery of the unchanged 3-oxobutanamides 1, the reaction was very complicated and none of the desired indolinones were detected. The formation of the Mn(III)-enolate complex with 1 would possibly be fast and successive electron transfer should easily occur,10a,11 so that the oxidation would cause a-cleavage of the amide bond to produce polymeric compounds.12 In order to recognize the oxidative radical reaction, the reaction was conducted in the presence of 1,1-diphenylethene as a radical trapping reagent, and 2-methyl-N,5,5-triphenyl-4,5-dihydrofuran-3-carboxamide VI could be obtained (Scheme 2 and also see the Experimental Section). Since the radical trapping product VI was confirmed, we investigated the reaction of 1b (R = Me) in neutral solvent, such as ethanol, to decrease the reaction rate.10a,13 Although the reaction did not proceed at room temperature for 2 days, the oxidation occurred at elevated temperature and the dimeric indolin-2-ones 3, 4, and 5 were obtained (Scheme 2). Unfortunately, dimerization of the indolinone intermediate 2b could not be controlled under the various reaction conditions.
The results suggested that the formation of the Mn(III)-enolate complex with both 1b and 2b was still fast in ethanol and the indolinone 2b was sufficiently reactive to dimerize under the stated conditions. However, we were able to isolate the cyclization products 3–5 even in low yields using the Mn(III)-based oxidation. In order to prevent the dimerization, we next explored the reaction using 3-oxo-N-phenylbutanamides bearing a substituent at the 2-position. We selected N,2-dimethyl-N-phenyl-3-oxobutanamide (6a), prepared by the methylation of 1b, and the reaction was carried out under the above conditions in ethanol, producing the desired 3-acetyl-1,3-dimethylindolin-2-one (7a) (Scheme 3). A stoichiometric amount of the oxidant was consumed within 12 minutes and the product 7a was obtained in 71% yield (Table 1, Entry 1). Surprisingly, separation using a neutral alumina column after the reaction led to the deacetylation product 8a (Entries 2, 3) (vide infra). The reaction in propanol did not proceed (Entry 4). However, when the reaction was conducted in glacial acetic acid, the oxidant was consumed within 3 minutes and the acetylindolinone 7a was quantitatively produced (Entry 5). The structure of 7a was easily characterized by disappearance of the quartet of methine proton and the collapsing of a doublet of the methyl group at the C-2 position of 6a to a singlet of the corresponding methyl group of 7a in the 1H NMR spectrum (see Experimental section).
With the efficient oxidative cyclization in hand, we applied the reaction to various substituted 3-oxobutanamides 6b–s in order to examine the substituent effect (Scheme 3 and Table 1, Entries 6-35). Introduction of a deactivating group toward an electrophile, such as a halogen atom in R1 on the aromatic ring, led to prolonging the reaction time (Table 1, Entries 6, 8, 9). On the other hand, an activating group in R1 tended to be similar or shortened the reaction time (Entries 10–12, 15, 16). In both cases, indolinones 7b–i were produced in high to quantitative yields. An N-alkyl or N-phenyl-substituent in R2 did not influence the cyclization, but all of 6j–m gave the corresponding indolinones 7j–m in high yields (Entries 17–20). When butanamide 6n bearing an ethyl group in R3 at the 2-position of the 3-oxobutanamide underwent the reaction under similar conditions, indolinone 7n was obtained in a moderate yield together with the unchanged 6n (Entry 21). Use of an excess amount of the oxidant led to the production of 7n in an almost quantitative yield (Entry 23). However, it took a longer reaction time to consume the oxidant. This tendency was also observed in the reaction of 6o (R3 = propyl), 6p (R3 = i-propyl), and 6q (R3 = butyl) (Entries 24–30). In the case of butylbutanamide 6q, the use of a large amount of the oxidant resulted in deacetylation followed by overoxidation to afford 3-acetoxy-3-butyl-1-methylindolin-2-one (9) (Entries 29, 30). N-Naphthylbutanamides 6r and 6s also produced the corresponding benzoindolinones 7r and 7s in excellent yields (Entries 33, 35).
Most of the reactions proceeded in acetic acid for 3–6 minutes using a stoichiometric amount of Mn(OAc)3 to give the corresponding indolinones 7. The plausible mechanism for the formation of 7 is outlined in Scheme 4. The rate-determining step would be the stage (6 → A) for the formation of the Mn(III)-enolate complex A.10a,11 Therefore, the bulky alkyl group of substituent R3 made the reaction rate slower and a steric hindrance derived from the bulkiness inhibited the cyclization affording the intermediate radical B. In order to accelerate the reaction, an excess amount of the oxidant would be necessary (Entries 23, 25, 27, 29).
We unexpectedly found the deacetylation during the separation and purification of the acetylindolinones 7 through a neutral alumina column (Table 1, Entries 2, 3, 7, 13, 14, 31). We were interested in the facile deacetylation affording indolinones 8, so that we scrutinized the reaction (Scheme 5). When acetylindolinone 7a (R1 = H) was treated in aqueous acetic acid, or acetic acid in the presence of Mn(OAc)2•4H2O, or in diethyl ether alone at 23 °C to reflux temperature, no reaction occurred and 7a was recovered unchanged (Table 2, Entries 1–3). However, when neutral alumina was added to 7a in diethyl ether and the mixture was stirred at room temperature in a flask, the deacetylation somewhat proceeded (Entry 4). After optimizing the reaction, a quantitative yield of 8a was achieved within 1 hour in diethyl ether (Entry 7). Methanol instead of diethyl ether as the solvent was not effective for the deacetylation (Entry 8). Other acetylindolinones 7b and 7g also underwent the deacetylation to give similar results (Entries 9, 10).
Although some methods are known for the transformation into indoles,14 we finally investigated the convenient route to substituted indoles from indolinones. With deacetylated indolinones 8 in hand, we examined the reduction of 8.15 The reaction using LiAlH4 (LAH) was carried out in dry tetrahydrofuran (THF) at 0 °C for 1 hour, then at room temperature for 4 hours, giving the desired indole 10a (Scheme 6). Since the reduction was quite easy, we tried to directly reduce the acetylindolinone 7a under the same conditions. Fortunately, the reduction efficiently proceeded and the desired indole 10a was produced in good yield. The reduction of the other indolinones 7b, g and 8b, g also gave similar results (Scheme 6).
CONCLUSION
We demonstrated the facile synthesis of 3-acetylindolin-2-ones 7 using a stoichiometric amount of Mn(OAc)3 in most cases, with a short reaction time and in high to quantitative yields, and also a simple retro-Claisen-like deacetylation of 7 by stirring with neutral alumina in diethyl ether at room temperature. In addition, both the 3-acetylindolinones 7 and the deacetylated 8 were easily transformed by normal LAH reduction into the corresponding indoles 10. Although some skillful methods for the synthesis of indolinones were recently reported,16 to the best of our knowledge, the Mn(III)-based oxidative cyclization of 3-oxobutanamides is one of the simplest and most convenient methods for the synthesis of substituted indolinone derivatives.
EXPERIMENTAL
Measurements. Melting points were taken using a Yanagimoto micromelting point apparatus and are uncorrected. The NMR spectra were recorded using a JNM AL300 or ECX 500 FT-NMR spectrometer at 300 or 500 MHz for 1H and at 75 or 125 MHz for 13C, with tetramethylsilane as the internal standard. The chemical shifts are reported in δ values (ppm) and the coupling constants in Hz. The IR spectra were measured in CHCl3 or KBr using a Shimadzu 8400 FT IR spectrometer and expressed in cm−1. The EI MS spectra were obtained by a Shimadzu QP-5050A gas chromatograph-mass spectrometer at the ionizing voltage of 70 eV. The high-resolution mass spectra and the elemental analyses were performed at the Instrumental Analysis Center, Kumamoto University, Kumamoto, Japan.
Materials. Manganese(II) acetate tetrahydrate, Mn(OAc)2•4H2O, was purchased from Wako Pure Chemical Ind., Ltd. Manganese(III) acetate dihydrate, Mn(OAc)3•2H2O, was prepared according to the modified method described in the literature (vide infra).17,18 Aniline and the substituted anilines from Wako Pure Chemical Ind., Ltd., naphthylamines from Kanto Chemical Co., Inc., and diketene and lithium aluminum hydride from Tokyo Kasei Co., Ltd., were purchased and used as received. The N-methylanilines were prepared by condensation of the corresponding anilines with paraformaldehyde in the presence of sodium methoxide in methanol followed by reduction with sodium borohydride. The other N-alkylanilines were purchased from Sigma-Aldrich Co., LLC. The N,2-dialkyl-3-oxobutanamides 6a-s were prepared by the reaction of neat N-alkylanilines with diketene followed by alkylation of the alkyl bromides in the presence of sodium hydride in dry tetrahydrofuran (THF). Flash column chromatography was performed on silica gel 60N (40-50 mm), which was purchased from Kanto Chemical Co., Inc., and thin layer chromatography (TLC) on Wakogel B-10 (45 mm) from Wako Pure Chemical Ind., Ltd. The activated neutral aluminum oxide (Al2O3) was purchased from Sigma-Aldrich Co., LLC.
Modified Preparation of Manganese(III) Acetate Dihydrate.17,18 To a 2 L round-bottomed flask, Mn(OAc)2•4H2O (66 g, 0.27 mol) and glacial AcOH (660 mL) were added and the mixture was heated at 90 °C to dissolve the components (normally for 10-30 min). The mixture must not be heated under reflux. After cooling, acetic anhydride (96 mL) was added to the mixture with stirring. Three portions of ground potassium permanganate, KMnO4, (11 g, 0.07 mol) were then slowly added with stirring. The mixture was heated under reflux for 1 h (Caution: the added KMnO4 must be dissolved in the reaction mixture. Otherwise the reaction mixture could be bumpy while cooling on the bench! Very dangerous!). After cooling, water (110 mL) was added and the mixture was kept at room temperature under dark conditions until the dark color of the supernatant solution turned transparent normally within for 2 weeks. The crystalline manganese(III) acetate formed was filtered, washed three times with glacial AcOH, washed twice with dried Et2O, and dried in a desiccator under reduced pressure using KOH as a drying agent, resulting in the bright-brown color of Mn(OAc)3•2H2O (67 g, 93% yield).
N,2-Dimethyl-3-oxo-N-phenylbutanamide (6a).16j,19 Yield 96%. Yellow liquid. IR (CHCl3): ν 1724 (C=O), 1651 (CONH) cm-1. 1H NMR (300 MHz, CDCl3): δ 7.49-7.36 (3H, m, arom H), 7.27-7.19 (2H, m, arom H), 3,41 (1H, q, J = 6.9 Hz, CH), 3.31 (3H, s, NMe), 2.02 (3H, s, Ac), 1.27 (3H, d, J = 6.9 Hz, Me) ppm. 13C NMR (75 MHz, CDCl3): δ 204.9 (C=O), 170.6 (N-C=O), 143.5, 130.1, 128.4, 127.5 (arom C), 51.6 (CH), 37.6 (N-Me), 27.9 (Ac), 13.9 (Me) ppm. HRMS (acetone/NBA) calcd for C12H16NO2 206.1181 (M+H). Found 206.1185.
N-(4-Fluorophenyl)-N,2-dimethyl-3-oxobutanamide (6b). Yield 92%. Orange liquid. IR (CHCl3): ν 1719 (C=O), 1653 (CONH) cm-1. 1H NMR (300 MHz, CDCl3): δ 7.22-7.10 (4H, m, arom H), 3.37 (1H, q, J = 6.9 Hz, CH), 3.28 (3H, s, N-Me), 2.03 (3H, s, Ac), 1.27 (3H, d, J = 6.9 Hz, Me) ppm. 13C NMR (75 MHz, CDCl3): δ 204.9 (C=O), 170.6 (N-C=O), 163.7, 160.4, 139.5, 129.5, 129.4, 117.2, 116.9 (arom C), 51.6 (CH), 37.8 (N-Me), 27.9 (Ac), 14.0 (Me) ppm. FAB HRMS (acetone/NBA) calcd for C12H15FNO2 224.1087 (M+H). Found 224.1089.
N-(4-Chlorophenyl)-N,2-dimethyl-3-oxobutanamide (6c). Yield 91%. Yellow liquid. IR (CHCl3): ν 1724 (C=O), 1655 (CONH) cm-1. 1H NMR (300 MHz, CDCl3): δ 7.44-7.41 (2H, m, arom H), 7.17-7.14 (2H, m, arom H), 3.38 (1H, q, J = 7.2 Hz, CH), 3.28 (3H, s, NMe), 2.05 (3H, s, Ac), 1.28 (3H, d, J = 7.2 Hz, Me) ppm. 13C NMR (75 MHz, CDCl3): δ 204.8 (C=O), 170.4 (N-C=O), 142.0, 134.3, 130.3, 128.9 (arom C), 51.6 (CH), 37.7 (N-Me), 27.9 (Ac), 14.0 (Me) ppm. HRMS (acetone/NBA) calcd for C12H15ClNO2 240.0791 (M+H). Found 240.0797.
N-(2-Chlorophenyl)-N,2-dimethyl-3-oxobutanamide (6d). Rotamer ratio = 1.28:1. Yield quant. Colorless liquid. IR (CHCl3): ν 1716 (C=O), 1655 (CONH) cm-1. 1H NMR (500 MHz, CDCl3): δ 7.58-7.53 (1H, m, arom H), 7.42-7.28 (3H, m, arom H), 3.26, 3.25 (3H, s, Me), 3.24-3.16 (1H, m, CH), 2.19, 2.01 (3H, s, Ac), 1.33, 1.23 (3H, d, J = 6.9 Hz, Me) ppm. 13C NMR (125 MHz, CDCl3): δ 205.0, 203.5 (C=O), 171.2, 170.1 (N-C=O), 140.33, 140.32, 132.9, 132.7, 130.9, 130.6, 130.0, 129.9, 129.7, 128.4 (arom C), 51.8, 51.4 (CH), 36.1, 36.0 (N-Me), 28.0, 27.6 (Ac), 13.9, 13.6 (Me) ppm. FAB HRMS (acetone/NBA) calcd for C12H15ClNO2 240.0791 (M+H). Found 240.0802.
N-(4-Methylphenyl)-N,2-dimethyl-3-oxobutanamide (6e).16j Yield 73%. Yellow liquid. IR (CHCl3): ν 1720 (C=O), 1651 (CONH) cm-1. 1H NMR (300 MHz, CDCl3): δ 7.27-7.23 (2H, m, arom H), 7.09-7.06 (2H, m, arom H), 3.41 (1H, q, J = 6.9 Hz, CH), 3.28 (3H, s, N-Me), 2.39 (3H, s, Me), 2.03 (3H, s, Ac), 1.25 (3H, d, J = 6.9 Hz, Me) ppm. 13C NMR (75 MHz, CDCl3): δ 204.9 (C=O), 170.8 (N-C=O), 140.1, 138.4, 130.7, 127.2 (arom C), 51.5 (CH), 37.7 (N-Me), 27.9 (Ac), 21.1 (Me), 13.9 (Me) ppm. FAB HRMS (acetone/NBA) calcd for C13H18NO2 220.1338 (M+H). Found 220.1337.
N-(2-Methylphenyl)-N,2-dimethyl-3-oxobutanamide (6f).16j Rotamer ratio = 1.24:1. Yield 84%. Brown liquid. IR (CHCl3): ν 1720 (C=O), 1651 (CONH) cm-1. 1H NMR (500 MHz, CDCl3): δ 7.33-7.24 (3H, m, arom H), 7.12-7.10 (1H, m, arom H), 3.29-3.18 (1H, m, CH), 3.23, 3,22 (3H, s, NMe), 2.28, 2.23 (3H, s, Me), 2.03, 2.00 (3H, s, Ac), 1.28, 1.26 (3H, d, J = 6.9 Hz, Me) ppm. 13C NMR (125 MHz, CDCl3): δ 205.0, 204.7 (C=O), 171.1, 170.5 (N-C=O), 141.8, 141.7, 135.9, 135.4, 131.9, 131.6, 128.8, 128.7, 128.5, 128.1, 127.6, 127.4 (arom C), 51.54, 51.52 (CH), 36.3 (N-Me), 27.9, 27.8 (Ac), 17.4, 17.3 (Me), 14.3, 14.1 (Me) ppm. Anal. Calcd for C13H17NO2: C, 71.21; H, 7.81; N, 6.39. Found: C, 71.18; H, 8.02; N, 6.27.
N-(4-Methoxylphenyl)-N,2-dimethyl-3-oxobutanamide (6g). Yield 62%. Orange liquid. IR (CHCl3): ν 1720 (C=O), 1651 (CONH) cm-1. 1H NMR (300 MHz, CDCl3): δ 7.14-7.09 (2H, m, arom H), 6.96-6.91 (2H, m, arom H), 3.82 (3H, s, OMe), 3.42 (1H, q, J = 6.9 Hz, CH), 3.27 (3H, s, N-Me), 2.03 (3H, s, Ac), 1.26 (3H, d, J = 6.9 Hz, Me) ppm. 13C NMR (75 MHz, CDCl3): δ 205.0 (C=O), 171.0 (N-C=O), 159.3, 136.2, 128.6, 115.1 (arom C), 55.5 (OMe), 51.5 (CH), 37.8 (N-Me), 28.0 (Ac), 13.9 (Me) ppm. FAB HRMS (acetone/NBA) calcd for C13H18NO3 236.1287 (M+H). Found 236.1288.
N-(3-Methoxyphenyl)-N,2-dimethyl-3-oxobutanamide (6h). Yield 86%. Yellow liquid. IR (CHCl3): ν 1720 (C=O), 1651 (CONH) cm-1. 1H NMR (300 MHz, CDCl3): δ 7.38-7.33 (1H, m, arom H), 6.94-6.90 (1H, m, arom H), 6.81-6.73 (2H, m, arom H), 3.83 (3H, s, OMe), 3.46 (1H, q, J = 6.0 Hz, CH), 3.30 (3H, s, NMe), 2.05 (3H, s, Ac), 1.27 (3H, d, J = 6.0 Hz, Me) ppm. 13C NMR (75 MHz, CDCl3): δ 204.9 (C=O), 170.6 (N-C=O), 160.7, 144.6, 130.8, 119.5, 113.7, 113.4 (arom C), 55.5 (OMe), 51.2 (CH), 37.5 (NMe), 28.0 (Ac), 14.0 (Me) ppm. HRMS (acetone/NBA) calcd for C13H18NO3 236.1287 (M+H). Found 236.1282.
N-(2-Methoxylphenyl)-N,2-dimethyl-3-oxobutanamide (6i). Rotamer ratio = 1.37:1. Yield 58%. Colorless microcrystals (from EtOH), mp 66-67 °C. IR (CHCl3): ν 1720 (C=O), 1651 (CONH) cm-1. 1H NMR (300 MHz, CDCl3): δ 7.38-7.34 (1H, m, arom H), 7.19-7.17 (1H, m, arom H), 7.16-6.98 (2H, m, arom H), 3.85, 3.84 (3H, s, OMe), 3.30-3.21 (1H, m, CH), 3.22, 3.21 (3H, s, N-Me), 2.12, 2.02 (3H, s, Ac), 1.23, 1.21 (3H, d, J = 6.0 Hz, Me) ppm. 13C NMR (75 MHz, CDCl3): δ 205.1, 204.6 (C=O), 171.8, 171.2 (N-C=O), 154.9, 131.8, 129.9, 129.8, 129.5, 128.9, 121.3, 112.0, 11.9 (arom C), 55.4, 55.2 (CH), 51.6 (OMe), 36.4, 36.3 (N-Me), 27.9, 27.2 (Ac), 14.0, 13.6 (Me) ppm. HRMS (acetone/NBA) calcd for C13H18NO3 236.1287 (M+H). Found 236.1289.
N-Ethyl-2-methyl-3-oxo-N-phenylbutanamide (6j).16o Yield 95%. Yellow liquid. IR (CHCl3): ν 1719 (C=O), 1647 (CONH) cm-1. 1H NMR (300 MHz, CDCl3): δ 7.49-7.37 (3H, m, arom H), 7.20-7.16 (2H, m, arom H), 3.78 (2H, q, J = 6.0 Hz, N-CH2-Me), 3.32 (1H, q, J = 6.9 Hz, CH), 2.02 (3H, s, Ac), 1.25 (3H, d, J = 6.9 Hz, Me), 1.15 (3H, t, J = 6.0 Hz, Me) ppm. 13C NMR (75 MHz, CDCl3): δ 204.9 (C=O), 170.1 (N-C=O), 141.9, 130.0, 128.6, 128.4 (arom C), 51.9 (CH), 44.4 (N-CH2), 27.9 (Ac), 13.8 (Me), 12.9 (Me) ppm. FAB HRMS (acetone/NBA) calcd for C13H18NO2 220.1338 (M+H). Found 220.1335.
N-Butyl-2-methyl-3-oxo-N-phenylbutanamide (6k). Yield 67%. Orange liquid. IR (CHCl3): ν 1718 (C=O), 1647 (CONH) cm-1. 1H NMR (300 MHz, CDCl3): δ 7.47-7.36 (3H, m, arom H), 7.19-7.15 (2H, m, arom H), 3.72 (2H, t, J = 6.0 Hz, N-CH2), 3.32 (1H, q, J = 6.0 Hz, CH), 2.01 (3H, s, Ac), 1.51 (2H, quint, J = 6.0 Hz, CH2), 1.33 (2H, sext, J = 6.0 Hz, CH2), 1.25 (3H, d, J = 6.0Hz, Me), 0.90 (3H, t, J = 6.0 Hz, Me) ppm. 13C NMR (75 MHz, CDCl3): δ 204.9 (C=O), 170.3 (N-C=O), 142.1, 130.0, 128.5, 128.4 (arom C), 51.9 (CH), 49.3 (N-CH2), 29.8 (CH2), 28.0 (Me), 20.0 (CH2), 13.9 (Me), 13.8 (Me ) ppm. FAB HRMS (acetone/NBA) calcd for C15H22NO2 248.1651 (M+H). Found 248.1642.
N-Isopropyl-2-methyl-3-oxo-N-phenylbutanamide (6l). Yield 90%. Yellow liquid. IR (CHCl3): ν 1719 (C=O), 1643 (CONH) cm-1. 1H NMR (300 MHz, CDCl3): δ 7.45-7.7.43 (3H, m, arom H), 7.27-7.12 (2H, m, arom H), 5.01 (1H, q, J = 6.9 Hz, N-CH), 3.17 (1H, q, J = 9.0 Hz, CH), 1.99 (3H, s, Ac), 1.23 (3H, d, J = 6.9 Hz, Me), 1.12 (3H, d, J = 7.2 Hz, Me), 1.04 (3H, d, J = 7.2 Hz, Me) ppm. 13C NMR (75 MHz, CDCl3): δ 205.0 (C=O), 170.0 (N-C=O), 138.2, 130.5, 130.4, 129.5 (arom C), 52.5 (CH), 46.3 (N-CH), 28.0 (Ac), 21.1, 20.8, 13.9 (Me) ppm. FAB HRMS (acetone/NBA) calcd for C14H20NO2 234.1494 (M+H). Found 234.1488.
2-Methyl-3-oxo-N,N-diphenylbutanamide (6m).20 Yield quant. Colorless prisms (from EtOH), mp 73-74 °C. IR (CHCl3): ν 1724 (C=O), 1664 (CONH) cm-1. 1H NMR (500 MHz, CDCl3): δ 7.44-7.19 (10H, m, arom H), 3.63 (1H, q, J = 7.0 Hz, CH), 2.07 (3H, s, Ac), 1.36 (3H, d, J = 7.0 Hz, Me) ppm. 13C NMR (125 MHz, CDCl3): δ 204.7 (C=O), 170.8 (>N-CO, 1C), 142.4, 130.1, 129.2, 128.9, 128.4, 126.3 (arom C), 52.4 (CH), 28.1 (Ac), 13.9 (Me) ppm.
2-Ethyl-N-methyl-3-oxo-N-phenylbutanamide (6n).19 Yield 48%. Yellow liquid. IR (CHCl3): ν 1717 (C=O), 1651 (CONH) cm-1. 1H NMR (300 MHz, CDCl3): δ 7.48-7.36 (3H, m, arom H), 7.20-7.17 (2H, m, arom H), 3.32 (3H, s, N-Me), 3.27 (1H, t, J = 6.0 Hz, CH), 2.06 (3H, s, Ac), 1.99-1.72 (2H, m, CH2-Me), 0.85 (3H, t, J = 7.2 Hz, Me) ppm. 13C NMR (75 MHz, CDCl3): δ 204.9 (C=O), 169.3 (N-C=O), 143.4, 130.0, 128.3, 127.7 (arom C), 59.2 (CH), 37.7 (N-Me), 28.1 (Ac), 23.0 (CH2), 12.3 (Me) ppm.
N-Methyl-3-oxo-N-phenyl-2-propylbutanamide (6o).19 Yield 47%. Brown liquid. IR (CHCl3): ν 1716 (C=O), 1651 (CONH) cm-1. 1H NMR (500 MHz, CDCl3): δ 7.46-7.43 (2H, m, arom H), 7.39-7.37 (1H, m arom H), 7.18-7.16 (2H, m, arom H), 3.36-3.33 (1H, m, CH), 3.31 (3H, s, N-Me), 2.02 (3H, s, Ac), 1.94-1.71 (1H, m, H-CH), 1.70-1.64 (1H, m, HC-H), 1.30-1.24 (1H, m, H-CH), 1.20-1.13 (1H, m, HC-H), 0.82 (3H, t, J = 7.5 Hz, Me) ppm. 13C NMR (125 MHz, CDCl3): δ 205.0 (C=O), 169.5 (N-C=O), 143.4, 130.0, 128.3, 127.7 (arom C), 57.6 (CH), 37.7 (N-Me), 31.7, 21.0 (CH2), 28.1 (Ac), 13.9 (Me) ppm.
2-Isopropyl-N-methyl-3-oxo-N-phenylbutanamide (6p). Yield 43%. Brown liquid. IR (CHCl3): ν 1717 (C=O), 1651 (CONH) cm-1. 1H NMR (500 MHz, CDCl3): δ 7.46-7.43 (2H, m, arom H), 7.39-7.36 (1H, m arom H), 7.13-7.11 (2H, m, arom H), 3.30 (3H, s, N-Me), 3.05 (1H, d, J = 10.5 Hz, CH), 2.50 (1H, m, CH), 2.17 (3H, s, Ac), 0.92 (3H, d, J = 7.0 Hz, Me), 0.75 (3H, d, J = 7.0 Hz, Me) ppm. 13C NMR (125 MHz, CDCl3): δ 205.0 (C=O), 168.7 (N-C=O), 143.3, 129.9, 128.2, 127.9 (arom C), 66.1 (CH), 37.8 (N-Me), 30.3 (Ac), 27.6 (CH), 21.0, 20.1 (Me) ppm. FAB HRMS (acetone/NBA) calcd for C14H20NO2 234.1494 (M+H). Found 234.1499.
2-Butyl-N-methyl-3-oxo-N-phenylbutanamide (6q).19 Yield 33%. Orange liquid. IR (CHCl3): ν 1720 (C=O), 1651 (CONH) cm-1. 1H NMR (500 MHz, CDCl3): δ 7.46-7.43 (2H, m, arom H), 7.39-7.36 (1H, m arom H), 7.18-7.16 (2H, m, arom H), 3.34-3.30 (1H, m, CH), 3.31 (3H, s, N-Me), 2.06 (3H, s, Ac), 1.93-1.87 (1H, m, H-CH), 1.73-1.68 (1H, m, HC-H), 1.26-1.18 (2H, m, CH2), 1.14-1.08 (2H, m, CH2), 0.85 (3H, t, J = 7.0 Hz, Me) ppm. 13C NMR (125 MHz, CDCl3): δ 205.0 (C=O), 169.5 (N-C=O), 143.4, 130.0, 128.3, 127.8 (arom C), 57.8 (CH), 37.7 (N-Me), 28.0 (Ac), 29.8, 29.3, 22.4 (CH2), 13.7 (Me) ppm.
N,2-Dimethyl-N-(1-naphthyl)-3-oxobutanamide (6r). Rotamer ratio = 1.2:1. Yield 77%. Colorless microcystals (from EtOH), mp 79 °C. IR (CHCl3): ν 1719 (C=O), 1651 (CONH) cm-1. 1H NMR (300 MHz, CDCl3): δ 7.92-7.31 (7H, m, arom H), 3.42 (3H, s, NMe), 3.18-3.13 (1H, m, CH), 1.97, 1.95 (3H, s, Ac), 1.26, 1.19 (3H, d, J = 6.9 Hz, Me) ppm. 13C NMR (75 MHz, CDCl3): δ 205.1, 203.8 (C=O), 171.9, 171.0 (N-C=O), 139.4, 139.2, 134.7, 134.6, 129.9, 129.8, 129.2, 129.0, 128.8, 128.7, 127.7, 127.6, 127.0, 126.9, 126.0, 125.8, 125.7, 125.5, 122.2, 121.8 (arom C), 51.7, 51.4 (CH), 37.2 (N-Me), 28.0, 27.8 (Ac), 14.2, 14.1 (Me) ppm. MS (rel intensity): m/z 255 (45), 157 (100), 128 (40), 112 (80), 70 (45). Anal. Calcd for C16H17NO2: C, 75.27; H, 6.71; N, 5.49. Found: C, 75.28; H, 6.92; N, 5.52.
N,2-Dimethyl-N-(2-naphthyl)-3-oxobutanamide (6s). Yield 79%. Colorless microcystals (from EtOH), mp 103 °C. IR (CHCl3): ν 1726 (C=O), 1654 (CONH) cm-1. 1H NMR (300 MHz, CDCl3): δ 7.96-7.85 (3H, m, arom H), 7.71 (1H, m, aron H), 7.56-7.53 (2H, m, arom H), 7.35-7.31 (1H, m, arom H), 3.49 (1H, q, J = 6.9 Hz, CH), 3.39 (3H, s, NMe), 2.00 (3H, s, Ac), 1.28 (3H, d, J = 6.9 Hz, Me) ppm. 13C NMR (75 MHz, CDCl3): δ 204.1 (C=O), 170.3 (N-C=O), 140.3, 133.1, 132.0, 129.9, 127.4, 127.3, 126.7, 126.6, 125.6, 124.6 (arom C), 51.3 (CH), 37.1 (N-Me), 27.6 (Ac), 13.3 (Me) ppm. MS m/z (rel intensity): 255 (55, M+), 157 (100), 127 (35), 112 (30). Anal. Calcd for C16H17NO2: C, 75.27; H, 6.71; N, 5.49. Found: C, 75.11; H, 6.91; N, 5.47.
Oxidation of 3-Oxo-N-phenylbutanamides 1a-c in Acetic Acid. To a 3-oxo-N-phenylbutanamide 1 (0.5 mmol) dissolved in glacial AcOH (15 mL) was added Mn(OAc)3•2H2O (1 or 2 mmol), and the mixture was degassed under reduced pressure for 30 min using an ultrasonicator for exchange with an argon atmosphere. The mixture was heated under reflux in an argon atmosphere until the brown color of Mn(III) disappeared (normally 1 or 2 min). The mixture was concentrated under reduced pressure and 2M HCl (20 mL) was added. The aqueous solution was then extracted three times with CHCl3 (20 mL x 3). The combined extracts were washed with a saturated aqueous solution of NaHCO3 and water, dried over anhydrous MgSO4, and then concentrated to dryness, giving an intractable mixture and no isolated products were obtained except for a small amount of unchanged 1.
Mn(III)-Based Oxidation of 3-Oxo-N-phenylbutanamide 1a (R = H) in the Presence of Alkene as a Radical Trapping Reagent.21 To a mixture of 3-oxobutanamide 1a (0.177 g; 1 mmol) and 1,1-diphenylethene (0.180 g; 1 mmol) in glacial AcOH (15 mL) was added Mn(OAc)3•2H2O (0.992 g; 4 mmol), then the mixture was heated under reflux until the brown color of Mn(III) disappeared (for 1 min). After the work-up described above, 2-methyl-N,5,5-triphenyl-4,5-dihydrofuran-3-carboxamide (VI) was obtained in 0.155 g (43% yield) as a colorless solid which was recrystallized using CHCl3–hexane.
2-Methyl-N,5,5-triphenyl-4,5-dihydrofuran-3-carboxamide (VI). Colorless needles (from CHCl3-hexane), mp 248-251 °C. IR (CHCl3): ν 3400-3200 (NH), 1662 (C=O), 1632 (C=C) cm-1. 1H NMR (500 MHz, CDCl3): δ 7.50-7.48 (2H, m, arom H), 7.42-7.40 (4H, m, arom H), 7.38-7.34 (4H, m, arom H), 7.31-7.26 (4H, m, arom H), 7.09-7.06 (1H, m, aron H), 6.84 (1H, s, NH), 3.68 (1H, s, CH2), 2.45 (3H, s, Me) ppm. 13C NMR (125 MHz, CDCl3): δ 163.6 (C=O), 152.6 (C-2), 144.9, 138.0 (arom C), 128.9, 128.5, 127.7, 125.7, 124.0, 120.0 (arom CH), 102.7 (C-3), 90.9 (C-5), 44.7 (C-4), 14.3 (Me) ppm. MS m/z (rel internsity): 355 (33, M+), 313 (40), 262 (100), 247 (70), 221 (45), 191 (90), 175 (40), 165 (75), 115 (70), 105 (3), 93 (60), 77 (70). Anal. Calcd for C24H21NO2•1/10H2O: C, 80.70; H, 5.93; N, 3.92. Found: C, 80.58; H, 5.96; N, 3.97.
Oxidation of N-Methyl-3-oxo-N-phenylbutanamide (1b) in Ethanol. A mixture of butanamide 1b (3 mmol) and Mn(OAc)3•2H2O (12 mmol) in EtOH (60 mL) was degassed under reduced pressure for 20 min using an ultrasonicator for exchange with an argon atmosphere and then heated under reflux until the brown color of Mn(III) disappeared (for 4 min). 2M HCl (25 mL) was added to the reaction mixture and the aqueous solution was extracted three times with CH2Cl2 (25 mL x 3). The combined extracts were washed with a saturated aqueous solution of NaHCO3, dried over anhydrous MgSO4, and then concentrated to dryness. The residue was separated on silica gel TLC (Wako B-10) while eluting with Et2O, affording the dimeric 3,3'-biindoline-2,2'-dione derivatives 3, 4, and 5. The analytical samples were further purified by recrystallization from the solvent specified in parentheses and their physical data are listed below.
3,3'-Diacetyl-1,1'-dimethyl-[3,3'-biindoline]-2,2'-dione (3). Yield 9%. Colorless microcrystals (from EtOH), mp 176-177 °C. IR (KBr): ν 1728 (C=O), 1686 (CONH) cm-1. 1H NMR (300 MHz, CDCl3): δ 7.31-7.26 (4H, m, arom H), 7.00-6.95 (2H, m arom H), 6.75-6.72 (2H, m, arom H), 3.19 (6H, s, N-Me), 2.10 (6H, s, Ac) ppm. 13C NMR (75 MHz, CDCl3): δ 199.3 (C=O), 171.9 (N-C=O), 144.4, 129.8, 127.2, 123.7, 122.4, 108.2 (arom C), 69.3 (>C<), 28.5 (N-Me), 26.7 (Ac) ppm. FAB HRMS (acetone/NBA) calcd for C22H21N2O4 377.1501 (M+H). Found 377.1517.
1,1'-Dimethyl-[3,3'-biindoline]-2,2'-dione (4). A 1:1 diastereomer mixture. Yield 7%. Colorless microcrystals (from EtOH), mp 197-198 °C (lit,22 mp 194-196 °C). IR (KBr): ν 1720 (CONH) cm-1. 1H NMR (300 MHz, CDCl3): δ 7.29-7.27 (1H, m, arom H), 7.11-7.08 (1H, m arom H), 6.95-6.90 (2H, m, arom H), 6.82-6.75 (3H, m, arom H), 6.70-6.67 (1H, m, arom H), 4.29 (1H, s, CH), 4.18 (1H, s, CH’), 3.28 (3H, s, N-Me), 3.12 (3H, s, N-Me’) ppm. 13C NMR (75 MHz, CDCl3): δ 175.9, 174.7 (N-C=O), 145.0, 144.2, 128.8, 128.4, 125.8, 124.8, 123.3, 123.2, 122.5, 122.4, 108.3, 108.0 (arom C), 46.2, 46.1 (CH), 26.3, 26.2 (N-Me) ppm. MS m/z (rel intensity): 292 (20, M+), 146 (100), 118 (18), 91 (35), 77 (12), 65 (12), 51 (13).
(E)-1,1'-Dimethyl-[3,3'-biindolinylidene]-2,2'-dione (5).23 Yield 32%. Reddish prisms (from EtOH), mp 275-276 °C (lit, mp 278 °C, 23a 274-275 °C23d). IR (KBr): ν 1680 (CONH) cm-1. 1H NMR (300 MHz, CDCl3): δ 9.20 (2H, d, J = 7.8 Hz, H-4 and H-4’), 7.4 (2H, t, J = 7.5 Hz, H-6 and H-6’), 7.07 (2H, t, J = 7.5 Hz, H-5 and H-5’), 6.79 (2H, d, J = 7.8 Hz, H-7 and H-7’), 3.29 (6H, s, N-Me) ppm. 13C NMR (75 MHz, CDCl3): δ 168.0 (N-C=O), 145.2, 132.4, 129.8, 122.4, 121.6, 107.7 (arom C), 26.1 (N-Me) ppm. MS m/z (rel intensity): 290 (100, M+), 262 (45), 233 (50), 218 (30), 146 (20), 117 (25).
Oxidation of N,2-Disubstituted N-Aryl-3-oxobutanamides 6a-s in Acetic Acid. The general procedure for the reaction of 3-oxobutanamides 6a-s with Mn(OAc)3•2H2O was as follows. To 3-oxobutanamide 6 (0.5 mmol) dissolved in glacial AcOH (15 mL) was added Mn(OAc)3•2H2O (1 mmol). The mixture was quickly heated under reflux using a pre-heated oil bath at 140 °C until the brown color of Mn(III) disappeared (normally within 3-5 min). The solvent was removed under reduced pressure and the residue was triturated with 2M HCl (15 mL). The aqueous mixture was extracted three times with CHCl3 (20 mL x 3), and the combined extracts were washed with a saturated aqueous solution of NaHCO3 and water, dried over anhydrous MgSO4, and then concentrated to dryness, giving the desired acetylindolinone 7. If needed, the obtained acetylindolinone 7 was purified by silica gel TLC eluting with Et2O–hexane (7:3 v/v) and the solid 7 was recrystallized from the appropriate solvent as mentioned below. When the separation of the product 7 was performed using neutral Al2O3 eluting with Et2O–hexane (7:3 v/v), deacetylated indolinone 8 was obtained (vide infra).
3-Acetyl-1,3-dimethylindolin-2-one (7a).16g,24 Yield quant. IR (CHCl3): ν 1720, 1709 (C=O) cm-1. 1H NMR (300 MHz, CDCl3): δ 7.35 (1H, t, J = 7.2 Hz, arom H), 7.16 (1H, d, J = 7.2 Hz, arom H), 7.09 (1H, t, J = 7.2 Hz, arom H), 6.92 (1H, d, J = 7.2 Hz, arom H), 3.30 (3H, s, Me), 1.97 (3H, s, Ac), 1.58 (3H, s, Me) ppm. 13C NMR (75 MHz, CDCl3): δ 201.0, 175.9 (C=O), 143.7, 129.4 (arom C), 129.2, 123.5, 123.3, 108.6 (arom CH), 62.0 (C-3), 26.6 (Me), 25.9 (Me), 18.9 (Me) ppm.
3-Acetyl-5-fluoro-1,3-dimethylindolin-2-one (7b). Yield 99%. Yellow liquid. IR (CHCl3): ν 1728, 1707 (C=O) cm-1. 1H NMR (500 MHz, CDCl3): δ 6.98 (1H, dt, J = 8.5, 2.5 Hz, arom H), 6.86 (1H, dd, J = 8.5, 2.5 Hz, arom H), 6.77 (1H, dd, J = 8.5, 4.0 Hz, arom H), 3.22 (3H, s, Me), 1.94 (3H, s, Me), 1.51 (3H, s, Me). 13C NMR (125MHz, CDCl3): δ 200.5, 175.4 (C=O), 159,4 (d, 1J = 242.2 Hz), 139.5, 130.8 (d, 3J = 8.3 Hz) (arom C), 115.4 (d, 2J = 22.8 Hz), 111.9 (d, 2J = 25.2 Hz), 109.0 (d, 3J = 8.4 Hz) (arom CH), 62.2 (C-3), 26.7, 25.9, 19.2 (Me). FAB HRMS (acetone/NBA) calcd for C12H13FNO2 222.0930 (M+H). Found 222.0929.
3-Acetyl-5-chloro-1,3-dimethylindolin-2-one (7c). Yield quant. Colorless microcrystals (from hexane), mp 80-83 °C. IR (CHCl3): ν 1728, 1713 (C=O) cm-1. 1H NMR (300 MHz, CDCl3): δ 7.33 (1H, dd, J = 8.1, 1.8, Hz, H-6), 7.16 (1H, d, J = 1.8 Hz, H-4), 6.84 (1H, d, J = 8.1 Hz, H-7), 3.23 (3H, s, Me), 2.02 (3H, s, Me), 1.58 (3H, s, Me). 13C NMR (75MHz, CDCl3): δ 200.3, 175.4 (C=O), 142.2, 130.9, 129.1 (arom C), 128.6, 124.2, 109.5 (arom CH), 62.0 (C-3), 26.8, 26.0, 19.3 (Me). Anal. Calcd for C12H12ClNO2: C, 60.49; H, 5.09; N, 5.89. Found: C, 60.41; H, 5.04; N, 5.96.
3-Acetyl-7-chloro-1,3-dimethylindolin-2-one (7d). Yield quant. Orange liquid. IR (CHCl3): ν 1717 (C=O) cm-1. 1H NMR (300 MHz, CDCl3): δ 7.29-7.26 (1H, m, arom H), 7.05-6.97 (2H, m, arom H), 3.66 (3H, s, Me), 1.99 (3H, s, Me), 1.57 (3H, s, Me). 13C NMR (75MHz, CDCl3): δ 200.3, 176.1 (C=O), 131.9 (arom C), 131.4, 124.0 (2C), 122.1 (arom CH), 115.9 (arom C), 61.7 (C-3), 30.0, 26.0, 19.5 (Me). FAB HRMS (acetone/NBA/NaI) calcd for C12H12ClNO2Na 260.0454 (M+Na). Found 260.0454.
3-Acetyl-1,3,5-trimethylindolin-2-one (7e). Yield quant. Colorless prisms (from EtOH), mp 92-95 °C (lit,16j,24 mp 90-93 °C). IR (CHCl3): ν 1724, 1701 (C=O) cm-1. 1H NMR (300 MHz, CDCl3): δ 7.16 (1H, dd, J = 7.5, 0.6, Hz, H-6), 6.94 (1H, d, J = 0.6 Hz, H-4), 6.80 (1H, d, J = 7.5 Hz, H-7), 3.28 (3H, s, Me), 2.33 (3H, s, Me), 1.94 (3H, s, Me), 1.58 (3H, s, Me). 13C NMR (75MHz, CDCl3): δ 201.2, 175.9 (C=O), 141.3, 132.3 (2C) (arom C), 129.4, 124.3, 108.6 (arom CH), 62.1 (C-3), 26.7, 25.9, 21.1, 18.9 (Me). Anal. Calcd for C13H15NO2: C, 71.87; H, 6.96; N, 6.45. Found: C, 71.74; H, 7.04; N, 6.55.
3-Acetyl-1,3,7-trimethylindolin-2-one (7f).24 Yield 94%. Colorless microcrystals (from EtOH), mp 80-83 °C (lit,16j mp 79-81 °C). IR (CHCl3): ν 1724, 1701 (C=O) cm-1. 1H NMR (300 MHz, CDCl3): δ 7.09-7.06 (1H, m, arom H), 6.97-6.95 (2H, m, arom H), 3.58 (3H, s, Me), 2.62 (3H, s, Me), 1.95 (3H, s, Me), 1.54 (3H, s, Me). 13C NMR (75MHz, CDCl3): δ 201.1, 176.6 (C=O), 141.5, 130.0, 120.2 (arom C), 132.8, 123.1, 121.4 (arom CH), 61.5 (C-3), 29.9, 25.8, 19.3, 19.0 (Me). Anal. Calcd for C13H15NO2: C, 71.87; H, 6.96; N, 6.45. Found: C, 71.73; H, 7.07; N, 6.37.
3-Acetyl-5-methoxy-1,3-dimethylindolin-2-one (7g). Yield 89%. Colorless microcrystals (from hexane), mp 89-92 °C. IR (CHCl3): ν 1724, 1701 (C=O) cm-1. 1H NMR (300 MHz, CDCl3): δ 6.88 (1H, dd, J = 8.4, 2.1, Hz, H-6), 6.826 (1H, d, J = 8.4 Hz, H-7), 6.764 (1H, d, J = 2.1 Hz, H-4), 3.80 (3H, s, MeO), 3.22 (3H, s, Me), 1.97 (3H, s, Me), 1.57 (3H, s, Me). 13C NMR (75MHz, CDCl3): δ 201.1, 175.6 (C=O), 156.5, 137.1, 130.6 (arom C), 113.7, 110.6, 109.0 (arom CH), 62.4 (C-3), 55.9 (MeO), 26.7, 25.9, 19.0 (Me). Anal. Calcd for C13H15NO3: C, 66.94; H, 6.48; N, 6.00. Found: C, 66.80; H, 6.59; N, 6.00.
A Mixture of 3-Acetyl-6-methoxy-1,3-dimethylindolin-2-one and 3-Acetyl-4-methoxy-1,3-dimethylindolin-2-one (7h). 6-Mthoxy-7h:4-methoxy-7h = 1.8:1. Yield 83%. Colorless microcrystals (from hexane), mp 100-103 °C. IR (CHCl3): ν 1730, 1701 (C=O) cm-1. 1H NMR (500 MHz, CDCl3): δ 7.35-7.30 (m, arom H), 7.04 (d, J = 8.0 Hz, arom H), 6.67 (d, J = 8.0 Hz, arom H), 6.61-6.58 (m, arom H), 6.52 (d, J = 1.5 H, arom H), 3.85, 3.82 (s, MeO), 3.28, 3.27 (s, Me), 1.95, 1.92 (s, Me), 1.61, 1.54 (s, Me). 13C NMR (75MHz, CDCl3): δ 201.1, 200.3, 176.3, 174.9 (C=O), 160.9, 155.6, 145.0, 144.9, 121.1, 116.2 (arom C), 130.3, 124.0, 106.9, 106.1, 101.7, 96.6 (arom CH), 61.5, 61.3 (C-3), 55.5, 55.4 (MeO), 26.8, 26.5, 25.6, 25.5, 18.8, 16.7 (Me). Anal. Calcd for C13H15NO3: C, 66.94; H, 6.48; N, 6.00. Found: C, 66.77; H, 6.54; N, 5.97.
3-Acetyl-7-methoxy-1,3-dimethylindolin-2-one (7i). Yield 89%. Brown liquid. IR (CHCl3): ν 1724, 1701 (C=O) cm-1. 1H NMR (300 MHz, CDCl3): δ 7.03 (1H, t, J = 8.1 Hz, H-5), 6.91 (1H, br. d, J = 8.1 Hz, H-6), 6.75 (1H, br. d, J = 7.8 Hz, H-4), 3.89 (3H, s, MeO), 3.56 (3H, s, Me), 1.95 (3H, s, Me), 1.54 (3H, s, Me). 13C NMR (75MHz, CDCl3): δ 201.0, 176.0 (C=O), 145.5, 131.5, 130.9, (arom C), 123.7, 116.0, 112.8 (arom CH), 62.2 (C-3), 56.0 (MeO), 29.9, 25.8, 19.0 (Me). FAB HRMS (acetone/NBA/NaI) calcd for C13H15NO3Na 256.0950 (M+Na). Found 256.0959.
3-Acetyl-1-ethyl-3-methylindolin-2-one (7j). Yield 96%. Orange liquid. IR (CHCl3): ν 1724, 1705 (C=O) cm-1. 1H NMR (300 MHz, CDCl3): δ 7.34 (1H, dd, J = 7.8, 1.2 Hz, arom H), 7.15 (1H, m, arom H), 7.08 (1H, br. t, J = 7.5 Hz, arom H9, 6.94 (1H, br. d, J = 7.8 Hz, arom H), 3.96-3.73 (2H, m, -CH2-CH3), 1.95 (3H, s, Me), 1.57 (3H, s, Me), 1.32 (3H, t, J = 7.2 Hz, -CH2-CH3). 13C NMR (75MHz, CDCl3): δ 201.0, 175.5 (C=O), 142.8, 129.7 (arom C), 129.1, 123.7, 123.0, 108.7 (arom CH), 62.0 (C-3), 35.0 (N-CH2), 25.8, 18.7 (Me), 12.5 (N-CH2-CH3). FAB HRMS (acetone/NBA/NaI) calcd for C13H15NO2Na 240.1000 (M+Na). Found 240.1004.
3-Acetyl-1-butyl-3-methylindolin-2-one (7k). Yield 98%. Orange liquid. IR (CHCl3): ν 1724, 1705 (C=O) cm-1. 1H NMR (300 MHz, CDCl3): δ 7.36-7.30 (1H, m, arom H), 7.16-7.14 (1H, m, arom H), 7.10-7.05 (1H, m, arom H), 6.92 (1H, br. d, J = 6.6 Hz, arom H), 3.87-3.69 (2H, m, N-CH2-CH2CH2CH3), 1.96 (3H, s, Me), 1.71 (2H, quint, J = 7.2 Hz, N-CH2-CH2-CH2CH3), 1.56 (3H, s, Me), 1.43 (2H, sex, J = 7.2 Hz, N-CH2CH2-CH2-CH3), 0.98 (3H, t, J = 7.2 Hz, N-CH2CH2CH2-CH3). 13C NMR (75MHz, CDCl3): δ 201.1, 175.8 (C=O), 143.2, 129.6 (arom C), 129.1, 123.7, 123.0, 108.9 (arom CH), 61.9 (C-3), 40.1 (N-CH2-CH2CH2CH3), 29.4 (CH2), 25.9 (Me), 20.2 (CH2), 18.9 (Me), 13.7 (Me). FAB HRMS (acetone/NBA/NaI) calcd for C15H19NO2Na 268.1313 (M+Na). Found 268.1312.
3-Acetyl-1-isopropyl-3-methylindolin-2-one (7l). Yield 93%. Orange liquid. IR (CHCl3): ν 1724, 1701 (C=O) cm-1. 1H NMR (500 MHz, CDCl3): δ 7.31 (1H, br. t, J = 8.0 Hz, arom H), 7.13 (1H, br. d, J = 7.0 Hz, arom H), 7.07 (2H, br. t, J = 7.5 Hz, arom H), 4.68 (1H, sep, J = 7.0 Hz, N-CHMe2), 1.93 (3H, s, Me), 1.55 (3H, s, Me), 1.54 (3H, d, J = 7.0 Hz, Me), 1.53 (3H, d, J = 7.0 Hz, Me). 13C NMR (75MHz, CDCl3): δ 200.9, 175.6 (C=O), 142.5, 129.8 (arom C), 128.8, 123.7, 122.7, 110.2 (arom CH), 61.9 (C-3), 44.2 (CH), 25.6 (Me), 19.3 (Me), 19.2 (Me), 18.7 (Me). FAB HRMS (acetone/NBA/NaI) calcd for C14H17NO2Na 254.1157 (M+Na). Found 254.1158.
3-Acetyl-3-methyl-1-phenylindolin-2-one (7m). Yield 91%. Colorless needles (from EtOH), mp 112-115 °C (lit,24d) mp 101-103 °C). IR (CHCl3): ν 1728, 1710 (C=O) cm-1. 1H NMR (500 MHz, CDCl3): δ 7.56 (2H, d, J = 7.5 Hz, arom H), 7.46-7.44 (3H, m, arom H), 7.28 (1H, t, J = 7.5 Hz, arom H), 7.22 (1H, d, J = 6.5 Hz, arom H), 7.13 (1H, t, J = 8.0 Hz, arom H), 6.90 (1H, d, J = 8.0 Hz, arom H), 2.11 (3H, s, Me), 1.70 (3H, s, Me). 13C NMR (125MHz, CDCl3): δ 200.7, 175.3 (C=O), 143.7, 134.1, 129.2 (arom C), 129.8, 129.1, 128.4, 126.4, 123.8, 123.7, 109.9 (arom CH), 62.1 (C-3), 26.0 (Me), 19.2 (Me). Anal. Calcd for C17H15NO2: C, 76.96; H, 5.70; N, 5.28. Found: C, 76.99; H, 5.68; N, 5.30.
3-Acetyl-3-ethyl-1-methylindolin-2-one (7n).16g Yield 98%. Orange liquid. IR (CHCl3): ν 1719, 1705 (C=O) cm-1. 1H NMR (300 MHz, CDCl3): δ 7.30-7.25 (1H, m, arom H), 7.10 (1H, d, J = 7.5 Hz, arom H), 7.03 (1H, t, J = 7.5 Hz, arom H), 6.83 (1H, d, J = 8.0 Hz, arom H), 3.22 (3H, s, Me), 2.21-2.07 (2H, m, CH2-CH3), 1.94 (3H, s, Me), 0.53 (3H, t, J = 7.5 Hz, CH2-CH3). 13C NMR (75MHz, CDCl3): δ 201.4, 175.0 (C=O), 144.3, 127.2 (arom C), 129.0, 123.9, 123.1, 108.3 (arom CH), 67.3 (C-3), 26.6 (Me x 2), 26.4 (CH2), 8.1 (Me). FAB HRMS (acetone/NBA/NaI) calcd for C13H15NO2Na 240.1000 (M+Na). Found 240.1000.
3-Acetyl-1-methy-3-propylindolin-2-one (7o). Yield 94%. Orange liquid. IR (CHCl3): ν 1722, 1705 (C=O) cm-1. 1H NMR (500 MHz, CDCl3): δ 7.35 (1H, t, J = 7.5 Hz, arom H), 7.17 (1H, d, J = 7.5 Hz, arom H), 7.10 (1H, t, J = 7.5 Hz, arom H), 6.90 (1H, d, J = 7.5 Hz, arom H), 3.29 (3H, s, Me), 2.20-2.08 (2H, m, CH2-CH2CH3), 2.00 (3H, s, Me), 1.05-0.97 (2H, m, CH2-CH2-CH3), 0.82 (3H, t, J = 6.0 Hz, CH2CH2-CH3). 13C NMR (125MHz, CDCl3): δ 201.3, 175.2 (C=O), 144.2, 127.6 (arom C), 129.0, 123.9, 123.1, 108.4 (arom CH), 66.8 (C-3), 35.5 (CH2), 26.5 (Me), 26.4 (Me), 17.2 (CH2), 14.1 (Me). FAB HRMS (acetone/NBA/NaI) calcd for C14H17NO2Na 254.1157 (M+Na). Found 254.1157.
3-Acetyl-3-isopropyl-1-methylindolin-2-one (7p). Yield 4%. Orange liquid. IR (CHCl3): ν 1722, 1707 (C=O) cm-1. 1H NMR (500 MHz, CDCl3): δ 7.34 (1H, dt, J = 7.5, 1.0 Hz, arom H), 7.28 (1H, d, J = 7.5 Hz, arom H), 7.10 (1H, t, J = 7.5 Hz, arom H9, 6.87 (1H, d, J = 7.5 Hz, arom H), 3.26 (3H, s, Me), 2.84 (1H, sep, J = 7.0 Hz, CHMe2), 2.17 (3H, s, Me), 0.89 (3H, d, J = 7.0 Hz, Me), 0.84 (3H, d, J = 7.0 Hz, Me). 13C NMR (125MHz, CDCl3): δ 202.3, 174.3 (C=O), 144.0, 129.5, (arom C), 128.7, 125.2, 122.8, 108.0 (arom CH), 70.4 (C-3), 34.5 (CH), 27.7 (Me), 26.3 (Me), 17.5 (Me), 17.1 (Me). FAB HRMS (acetone/NBA/NaI) calcd for C14H18NO2 232.1338 (M+H). Found 232.1335.
3-Acetyl-3-butyl-1-methylindolin-2-one (7q). Yield 81%. Colorless liquid. IR (CHCl3): ν 1722, 1705 (C=O) cm-1. 1H NMR (500 MHz, CDCl3): δ 7.35 (1H, t, J = 7.5 Hz, arom H), 7.17 (1H, d, J = 7.5 Hz, arom H), 7.104 (1H, t, J = 7.5 Hz, arom H), 6.91 (1H, d, J = 7.5 Hz, arom H), 3.30 (3H, s, Me), 2.22-2.11 (2H, m, CH2-CH2CH2CH3), 2.00 (3H, s, Me), 1.29-1.17 (2H, m, CH2 x 2), 1,00-0.91 (1H, m, CHH), 0.78 (3H, t, J = 7.5 Hz, N-CH2CH2CH2-CH3), 0.75-0.72 (1H, m, CHH). 13C NMR (125MHz, CDCl3): δ 201.4, 175.2 (C=O), 144.2, 127.6 (arom C), 129.0, 123.9, 123.2, 108.4 (arom CH), 66.7 (C-3), 33.1 (N-CH2-CH2CH2CH3), 26.5 (Me), 26.4 (Me), 25.8 (CH2), 22.7 (CH2), 13.8 (Me). FAB HRMS (acetone/NBA/NaI) calcd for C15H19NO2Na 268.1313 (M+Na). Found 268.1316.
3-Acetoxy-3-butyl-1-methylindolin-2-one (9). Yield 60%. Colorless liquid. IR (CHCl3): ν 1728 (C=O), 1244 (C-O-C) cm-1. 1H NMR (500 MHz, CDCl3): δ 7.32 (1H. t, J = 7.5 Hz, arom H), 7.21 (1H, d, J = 7.5 Hz, arom H), 7.05 (1H, t, J = 7.5 Hz, arom H), 6.84 (1H, d, J = 7.5 Hz, arom H), 3.29 (3H, s, Me), 2.04 (3H, s, Me), 2.00-1.90 (2H, m, N-CH2-CH2CH2CH3), 1.29-1.17 (3H, m, CHH-CH2CH3), 1.14-1.08 (1H, m, CHH-CH2CH3), 0.83 (3H, t, J = 7.5 Hz, N-CH2CH2CH2-CH3). 13C NMR (125MHz, CDCl3): δ 175.2, 169.0 (C=O), 144.0, 127.9 (arom C), 129.6, 122.6, 122.5 108.3 (arom CH), 80.1 (C-3), 36.4 (N-CH2-CH2CH2CH3), 26.4 (Me), 24.1 (CH2), 22.7 (CH2), 20.7 (Me), 13.8 (Me). FAB HRMS (acetone/NBA/NaI) calcd for C15H19NO3Na 284.1263 (M+Na). Found 284.1254.
3-Acetyl-1,3-dimethyl-1H-benzo[g]indol-2(3H)-one (7r). Yield 96%. Colorless microcrystals (from EtOH), mp 128 °C. IR (CHCl3): ν 1724, 1647 (C=O) cm-1. 1H NMR (300 MHz, CDCl3): δ 7.79 ( 1H, d, J = 8.0 Hz, arom H), 7.58 (1H, d , J = 8.0 Hz, arom H), 7.50 (2H, t, J = 7.5 Hz, arom H), 7.15 (1H, d, J = 8.0 Hz, arom H), 7.07 (1H, d, J = 8.0 Hz, arom H), 3.60 (3H, s, Me), 2.05 (3H, s, Me), 1.78 (3H, s, Me). 13C NMR (75MHz, CDCl3): δ 201.3, 169.5 (C=O), 136.4, 133.7, 133.0, 118.8 (arom C), 127.2, 127.1, 126.8, 123.5, 123.0, 109.2 (arom CH), 60.9 (C-3), 29.6 (Me), 27.1 (Me), 26.9 (Me). Anal. Calcd for C16H15NO2: C, 75.87; H, 5.97; N, 5.53. Found: C, 75.83; H, 6.11; N, 5.60.
1-Acetyl-1,3-dimethyl-1H-benzo[e]indol-2(3H)-one (7s). Yield 99%. Colorless microcrystals (from EtOH), mp 132 °C. IR (CHCl3): ν 1726, 1694 (C=O) cm-1. 1H NMR (300 MHz, CDCl3): δ 7.83 (1H, d, J = 8.4 Hz, arom H), 7.75 (1H, d, J = 8.1 Hz, arom H), 7.48 (1H, d, J = 8.1 Hz, arom H), 7.35 (1H, t, J = 8.1 Hz, arom H), 7.24 (1H, t, J = 8.1 Hz, arom H), 7.17 (1H, d, J = 8.4 Hz, arom H), 3.30 (3H, s, Me), 1.74 (3H, s, Me), 1.66 (3H, s, Me). 13C NMR (75MHz, CDCl3): δ 201.0, 176.0 (C=O), 141.6, 130.4 (2C), 128.9 (arom C), 130.3, 129.3, 127.8, 124.0, 121.3, 109.5 (arom CH), 63.0 (C-3), 26.7 (Me), 25.7 (Me), 18.4 (Me). Anal. Calcd for C16H15NO2: C, 75.87; H, 5.97; N, 5.53. Found: C, 75.98; H, 6.14; N, 5.77.
Deacetylation of 3-Actyl-1,3-dimethylindolin-2-ones 7. To the obtained dimethylindolinone 7 (0.5 mmol) in Et2O (30 mL) was added neutral Al2O3 (7 g) in a 100-mL round-bottomed flask, and stirred at room temperature for 1 h. The reaction mixture was filtered using a 3G2 glass filter, washed with CHCl3, and concentrated. The residue was again dissolved in CHCl3, washed with a saturated aqueous solution of NaHCO3, water, dried over anhydrous MgSO4, and then concentrated to dryness. The crude deacetylated indolinone 8 was separated by silica gel TLC eluting with hexane. The solid product 8 was recrystallized from the appropriate solvent as mentioned below.
1,3-Dimethylindolin-2-one (8a).16a,16d,16n,25 Yield quant. Colorless prisms (from EtOH), mp 152 °C. IR (CHCl3): ν 1716 (C=O) cm-1. 1H NMR (300 MHz, CDCl3): δ 7.29 (1H, td, J = 7.5, 0.9 Hz, arom H), 7.24 (1H, d, J = 7.8 Hz, arom H), 7.06 (1H, dt, J = 7.5, 0.9 Hz, arom H), 6.83 (1H, d, J = 7.8 Hz, arom H), 3.44 (1H, q, J = 7.8 Hz, >CH-), 3.21 (3H, s, Me), 1.48 (3H, d, J = 7.8 Hz, Me) ppm. 13C NMR (75 MHz, CDCl3): δ 178.7 (C=O), 143.9, 130.6 (arom C), 127.8, 123.4, 122.3, 107.9 (arom CH), 40.5 (C-3), 26.1 (Me), 15.3 (Me) ppm. MS m/z (rel intensity) 161 (M+, 70), 146 (45), 132 (10), 118 (100), 91 (20).
5-Fluoro-1,3-dimethylindolin-2-one (8b).16d Yield 72%. 1H NMR (500 MHz, CDCl3): δ 6.99-6.95 (2H, m, arom H), 6.75-6.73 (1H, m, arom H), 3.42 (1H, q, J = 7.5 Hz, >CH-), 3.23 (3H, s, Me), 1.47 (3H, d, J = 7.5 Hz, Me) ppm. 13C NMR (125 MHz, CDCl3): δ 178.0 (C=O), 159.1 (d, J = 238.5 Hz), 139.8, 132.1 (d, J = 8.4 Hz) (arom C), 113.7 (d, J = 23.9 Hz), 111.5 (d, J = 23.9 Hz), 108.2 (d, J = 8.4 Hz) (arom CH), 40.7 (C-3), 26.2 (Me), 15.1 (Me) ppm.
5-Chloro-1,3-dimethylindolin-2-one (8c).26 Yield 31%. Colorless prisms (from CHCl3-hexane), mp 66 °C. IR (CHCl3): ν 1705 (C=O) cm-1. 1H NMR (300 MHz, CDCl3): δ 7.249 (1H, dd, J = 8.1, 2.4 Hz, arom H), 7.25 (1H, d, J = 2.4 Hz, arom H), 6.74 (1H, d, J = 8.1 Hz, arom H), 3.42 (1H, q, J = 7.8 Hz, >CH-), 3.19 (3H, s, Me), 1.47 (3H, d, J = 7.8 Hz, Me) ppm. 13C NMR (75 MHz, CDCl3): δ 178.0 (C=O), 142.5, 132.2, 127.7 (arom C), 127.7, 124.0, 108.8 (arom CH), 40.6 (C-3), 26.3 (Me), 15.2 (Me) ppm. MS m/z (rel intensity) 197 (M+, 30), 195 (M+, 100), 180 (30), 160 (95), 152 (50), 117 (95), 89 (30). Anal. Calcd for C10H10ClNO: C, 61.39; H, 5.15; N, 7.16. Found: C, 61.22; H, 5.04; N, 7.05.
5-Methoxy-1,3-dimethylindolin-2-one (8g).16d,27 Yield 96%. Yellow liquid. IR (CHCl3): ν 1697 (C=O) cm-1. 1H NMR (300 MHz, CDCl3): δ 6.86 (1H, m, arom H), 6.80 (1H, dd, J = 8.5, 2.5 Hz, arom H), 6.72 (1H, d, J = 8.5, arom H), 3.79 (3H, s, MeO), 3.39 (1H, q, J = 7.5 Hz, >CH-), 3.14 (3H, s, Me), 1.46 (3H, d, J = 7.5 Hz, Me) ppm. 13C NMR (75 MHz, CDCl3): δ 178.2 (C=O), 155.9, 137.5, 132.0 (arom C), 111.9, 111.2, 108.1 (arom CH), 55.8 (MeO), 40.9 (C-3), 26.2 (Me), 15.4 (Me) ppm.
4-Methoxy-1,3-dimethylindolin-2-one (8h).28 Yield 76%. Colorless prisms (from CHCl3-hexane), mp 70 °C. IR (CHCl3): ν 1705 (C=O) cm-1. 1H NMR (300 MHz, CDCl3): δ 7.22 (1H, t, J = 8.4 Hz, arom H), 6.62 (1H, d, J = 8.4 Hz, arom H), 6.47 (1H, d, J = 8.4 Hz, arom H), 3.84 (3H, s, MeO), 3.43 (1H, q, J = 7.5 Hz, >CH-), 3.16 (3H, s, Me), 1.49 (3H, d, J = 7.5 Hz, Me) ppm. 13C NMR (75 MHz, CDCl3): δ 178.6 (C=O), 155.6, 144.8, 116.16 (arom C), 128.8, 105.3, 101.1 (arom CH), 55.0 (MeO), 39.3 (C-3), 26.0 (Me), 14.0 (Me) ppm. MS m/z (rel intensity) 191 (M+, 80), 176 (100), 148 (60), 133 (50), 117 (30), 105 (20), 91 (35), 77 (50).
1,3-Dimethyl-1H-benzo[g]indol-2(3H)-one (8r). Yield 63%. Colorless prisms (from CHCl3-hexane), mp 80-81 °C. IR (CHCl3): ν 1665 (C=O) cm-1. 1H NMR (300 MHz, CDCl3): δ 7.71 (1H, d, J = 8.1 Hz, arom H), 7.52-7.40 (3H, m, arom H), 7.31 (1H, d, J = 7.2 Hz, arom H), 6.94 (1H, d, J = 7.5 Hz, arom H), 4.14 (1H, q, J = 7.5 Hz, >CH-), 3.50 (3H, s, Me), 1.65 (3H, d, J = 7.5 Hz, Me) ppm. 13C NMR (75 MHz, CDCl3): δ 171.5 (C=O), 137.4, 135.4, 133.3, 119.3 (arom C), 126.9, 126.4, 125.8, 123.7, 122.3, 108.5 (arom CH), 41.2 (C-3), 29.3 (Me), 24.3 (Me) ppm. MS m/z (rel intensity) 211 (M+, 100), 196 (50), 168 (80), 133 (50), 127 (30), 83 (25). FAB HRMS (acetone/NBA) calcd for C14H13NO 211.0997 (M). Found 211.0984.
Reduction of Indolinones 7 and 8. Acetylindolinone 7 or deacetylated indolinone 8 (0.2 mmol) was dissolved in dry THF (4 mL) and cooled at 0 °C. LiAlH4 (0.4 mmol) was added and the mixture was stirred at 0 °C for 1 h, then at room temperature for 4 h under an argon atmosphere. The reaction was quenched by adding EtOAc (1 mL) and a saturated aqueous solution of Rochelle salt (2 mL). The aqueous solution was filtered through a Celite column, washed with EtOAc, and the filtrate then concentrated dryness. The residue was separated by silica gel TLC eluting with hexane-EtOAc (95:5 v/v), giving the corresponding indole 10.
1,3-Dimethyl-1H-indole (10a).15,29 Yield 86%. Yellow liquid. IR (CHCl3): ν 1616 (C=C) cm-1. 1H NMR (500 MHz, CDCl3): δ 7.56 (1H, d, J = 8.5 Hz, arom H), 7.27 (1H, d, J = 8.5 Hz, arom H), 7.21 (1H, t, J = 7.3 Hz, arom H), 7.10 (1H, t, J = 7.3 Hz, arom H), 6.82 (1H, s, H-2), 3.73 (3H, s, Me), 2.32 (3H, s, Me) ppm. 13C NMR (125 MHz, CDCl3): δ 136.9, 128.6, 110.1 (arom C), 126.5, 121.4, 118.9, 118.4, 109.0 (arom CH), 32.5 (Me), 9.5 (Me) ppm.
5-Fluoro-1,3-dimethyl-1H-indole (10b).30 Yield 69%. Yellow liquid. IR (CHCl3): ν 1625 (C=C) cm-1. 1H NMR (500 MHz, CDCl3): δ 7.18 (1H, dd, J = 10.0, 2.5 Hz, arom H), 7.14 (1H, dd, J = 10.0, 4.0 Hz, arom H), 6.94 (1H, dt, J = 10.0, 2.5 Hz, arom H), 6.83 (1H, s, H-2), 3.69 (3H, s, Me), 2.26 (3H, s, Me) ppm. 13C NMR (125 MHz, CDCl3): δ 157.5 (d, J = 234.0 Hz), 133.6 (s), 128.8 (d, J = 9.7 Hz), 109.9 (d, J = 4.8 Hz) (arom C), 128.2 (s), 109.7 (d, J = 15.6 Hz), 109.5 (s), 103.7 (d, J = 22.9 Hz) (arom CH), 32.7 (Me), 9.4 (Me) ppm.
5-Methoxy-1,3-dimethyl-1H-indole (10g).30 Yield 79%. IR (CHCl3): ν 1620 (C=C) cm-1. 1H NMR (500 MHz, CDCl3): δ 7.07 (1H, d, J = 8.5 Hz, arom H), 6.92 (1H, d, J = 2.5 Hz, arom H), 6.79 (1H, dd, J = 8.5, 2.5 Hz, arom H), 6.70 (1H, s, arom H), 3.79 (3H, s, Me), 3.60 (3H, s, Me), 2.21 (3H, s, Me) ppm. 13C NMR (125 MHz, CDCl3): δ 153.6, 132.4, 128.9, 109.4 (arom C), 127.2, 111.6, 109.7, 100.8 (arom CH), 55.9 (Me), 32.6 (Me), 9.5 (Me) ppm.
ACKNOWLEDGEMENTS
This research was supported by a Grant-in-Aid for Scientific Research (C), No. 25410049, from the Japan Society for the Promotion of Science, and also by the 2013-Core Research Program, “Integrated Science for Molecular Chirality in Biology and Chemistry,” Graduate School of Science and Technology, Kumamoto University, Japan. We gratefully acknowledge Nissan Chemical Industries, Ltd., for financial support. HN thanks Mr. Shin-tarou Hashimoto and Mr. Shin-ji Tadano, Department of Science, Faculty of Science, Kumamoto University, Japan, for their technical assistance.
References
1. a) J. A. Joule and K. Mills, 'Heterocyclic Chemistry,' 5th ed., Wiley, Chichester, 2010; b) R. J. Sundberg, 'The Chemistry of Indoles,' Academic Press, Inc., New York, 1970; c) B. Robinson, 'Fischer Indole Synthesis,' Wiley, Chichester, 1982; d) R. J. Sundberg, 'Indoles,' Academic Press, Inc., London, 1996; e) D. L. Hughes, Org. Prep. Proc. Int., 1993, 25, 607. CrossRef
2. a) U. Pindur and H. Erfanian-Abdoust, Chem. Rev., 1989, 89, 1681; CrossRef b) G. R. Humphrey and J. T. Kuethe, Chem. Rev., 2006, 106, 2875; CrossRef c) S. Cacchi and G. Fabrizi, Chem. Rev., 2005, 105, 2873; CrossRef d) M. Shiri, Chem. Rev., 2012, 112, 3508; CrossRef e) A. W. Schmidt, K. R. Reddy, and H.-J. Knölker, Chem. Rev., 2012, 112, 3193; CrossRef f) G. H. Kirsch, Curr. Org. Chem., 2001, 5, 507. CrossRef
3. a) S. Tadano, Y. Mukaeda, and H. Ishikawa, Angew. Chem. Int. Ed., 2013, 52, 7990; CrossRef b) E. D. Styduhar, A. D. Huters, N. A. Weires, and N. K. Garg, Angew. Chem. Int. Ed., 2013, 52, 1; CrossRef c) G. G. A. Cordell, 'The Alkaloids: Chemistry and Biology,' Vol. 60, Elsevier, San Diego, 2003; d) W. Fröhner, M. P. Krahl, K. R. Reddy, and H.-J. Knölker, Heterocycles, 2004, 63, 2393. CrossRef
4. a) G. A. Cordell and J. E. Saxton, 'The Alkaloids: Chemistry and Physiology,' Vol. 20, Academic Press, Inc., New York, 1981, pp. 3–295; b) T. Hino and M. Nakagawa, 'The Alkaloids: Chemistry and Pharmacology,' Vol. 34, ed. by A. Brossi, Academic Press, Inc., New York, 1989, pp. 1–75; c) U. Pindur and T. Lemster, Recent Res. Dev. Org. Bioorg. Chem., 1997, 1, 33.
5. a) T. Tsuchimoto, H. Matsubayashi, M. Kaneko, Y. Nagase, T. Miyamura, and E. Shirakawa, J. Am. Chem. Soc., 2008, 130, 15823; CrossRef b) Y. Ooyama, Y. Shimada, Y. Kagawa, I. Imae, and Y. Harima, Org. Biomol. Chem., 2007, 5, 2046; CrossRef c) K. Kawaguchi, K. Nakano, and K. Nozaki, J. Org. Chem., 2007, 72, 5119; CrossRef d) N.-K. Kim, K.-J. Chang, D. Moon, M. S. Jah, and K.-S. Jeong, Chem. Commun., 2007, 3401. CrossRef
6. a) Z.-Q. Cong and H. Nishino, Synthesis, 2008, 2686; b) Z.-Q. Cong and H. Nishino, Heterocycles, 2009, 78, 397. CrossRef
7. a) H. Nishino, H. Kamachi, H. Baba, and K. Kurosawa, J. Org. Chem., 1992, 57, 3551; CrossRef b) Z.-Q. Cong, T. Miki, O. Urakawa, and H. Nishino, J. Org. Chem., 2009, 74, 3978; CrossRef c) Y. Maemura, Y. Tanoue, and H. Nishino, Heterocycles, 2012, 85, 2491. CrossRef
8. a) T. Tsubusaki and H. Nishino, Heterocycl. Commun., 2009, 15, 79; CrossRef b) T. Tsubusaki and H. Nishino, Tetrahedron, 2009, 65, 9448. CrossRef
9. K. Kurosawa, Bull. Chem. Soc. Jpn., 1969, 42, 1456. CrossRef
10. a) B. B. Snider, Chem. Rev., 1996, 96, 339; CrossRef b) G. G. Melikyan, Org. React., 1997, 49, 427; c) A. S. Demir and M. Emrullahoglu, Curr. Org. Synth., 2007, 4, 321; CrossRef d) X.-Q. Pan, J.-P. Zou, and W. Zhang, Mol. Divers., 2009, 13, 421; CrossRef e) K. Asahi and H. Nishino, Tetrahedron, 2008, 64, 1620; CrossRef f) Y. Ito, S. Jogo, N. Fukuda, R. Okumura, and H. Nishino, Synthesis, 2011, 1365; g) H. Nishino, R. Kumabe, R. Hamada, and M. Yakut, Tetrahedron, 2014, 70, 1437. CrossRef
11. B. B. Snider, Tetrahedron, 2009, 65, 10738. CrossRef
12. a) I. H. Bowen, P. Gupta, M. S. Khan, and J. R. Lewis, J. Chem. Soc., Perkin Trans. 1, 1972, 2554; b) B. Rindone and C. Scolastico, Tetrahedron Lett., 1974, 15, 3379; CrossRef c) G. Galliani, B. Rindone, and C. Scolastico, Tetrahedron Lett., 1975, 16, 1285; CrossRef d) H. Nishino and K. Kurosawa, Bull. Chem. Soc. Jpn., 1983, 56, 1682. CrossRef
13. K. Asahi and H. Nishino, Synthesis, 2009, 409.
14. a) J. E. Thomson, A. F. Kyle, K. B. Ling, S. R. Smith, A. M. Z. Slawin, and A. D. Smith, Tetrahedron, 2010, 66, 3801; CrossRef b) M. M. Bostos, L. M. U. Mayer, E. C. S. Figueira, M. Soares, W. B. Kover, and N. Boechat, J. Heterocycl. Chem., 2008, 45, 969; CrossRef c) S. J. Garden, R. B. de Silva, and A. C. Pinto, Tetrahedron, 2002, 58, 8399; CrossRef d) W. Wierenga, J. Griffin, and M. A. Warpehoski, Tetrahedron Lett., 1983, 24, 2437; CrossRef e) A. Kubo and T. Nakai, Synthesis, 1980, 365. CrossRef
15. P. L. Julian and H. C. Printy, J. Am. Chem. Soc., 1949, 71, 3206. CrossRef
16. a) B. Li, Y. Park, and S. Chang, J. Am. Chem. Soc., 2014, 136, 1125; CrossRef b) Y. Yang, J. Han, X. Wu, S. Mao, J. Yu, and L. Wang, Synlett, 2014, 25, 1419; CrossRef c) J.-H. Fan, M.-B. Zhou, Y. Liu, W.-T. Wei, X.-H. Ouyang, R.-J. Song, and J.-H. Li, Synlett, 2014, 25, 657; CrossRef d) C. Liu, D. Liu, W. Zhang, L. Zhou, and A. Lei, Org. Lett., 2013, 15, 6166; CrossRef e) J. Wang, Y. Yuan, R. Xiong, D. Zhang-Negrerie, Y. Du, and K. Zhao, Org. Lett., 2012, 14, 2210; CrossRef f) D. Qian and J. Zhang, Chem. Commun., 2012, 48, 7082; CrossRef g) X. Ju, Y. Liang, P. Jia, W. Li, and W. Yu, Org. Boimol. Chem., 2012, 10, 4981; CrossRef h) W.-W. Chan, T.-L. Kwong, and W.-Y. Yu, Org. Boimol. Chem., 2012, 10, 3749; CrossRef i) H.-L. Wang, Z. Li, G.-W. Wang, and S.-D. Yang, Chem. Commun., 2011, 47, 11336; CrossRef j) Z. Yu, L. Ma, and W. Yu, Synlett, 2010, 17, 2607; CrossRef k) B. Zaleska and S. Lis, Synth. Commun., 2001, 31, 189; CrossRef l) B. S. Gerstenberger, J. Lin, Y. S. Mimieux, L. E. Brown, A. G. Oliver, and J. P. Konopelski, Org. Lett., 2008, 10, 369; CrossRef m) B. Lu and D. Ma, Org. Lett., 2006, 8, 6115; CrossRef n) C. Leroi, D. Bertin, P.-E. Dufils, D. Gigmes, S. Marque, P. Tordo, J.-L. Couturier, O. Guerret, and M. A. Ciufolini, Org. Lett., 2003, 5, 4943; CrossRef o) D. Li and W. Yu, Adv. Synth. Catal., 2013, 355, 3708. CrossRef
17. P. J. Andrulis, Jr., M. J. S. Dewar, R. Dietz, and R. L. Hunt, J. Am. Chem. Soc., 1966, 88, 5473. CrossRef
18. E. I. Heiba, R. M. Dessau, and W. J. Koehl, Jr., J. Am. Chem. Soc., 1969, 91, 138. CrossRef
19. D. J. Cook and W. C. Lawall, J. Am. Chem. Soc., 1948, 70, 1918. CrossRef
20. M. Sato, H. Ogasawara, S. Komatsu, and T. Kato, Chem. Pharm. Bull., 1984, 32, 3848. CrossRef
21. C.-Y. Qian, H. Nishino, and K. Kurosawa, Bull. Chem. Soc. Jpn., 1991, 64, 3557. CrossRef
22. T. Hino, Chem. Pharm. Bull., 1961, 9, 979. CrossRef
23. a) Y. K. Voronina, D. B. Krivolapov, A. V. Bogdanov, V. F. Mironov, and I. A. Litvinov, J. Struct. Chem., 2012, 53, 413; CrossRef b) J. Bergman and I. Romero, J. Heterocycl. Chem., 2010, 47, 1215; CrossRef c) A. V. Bogdanov, V. F. Mironov, L. I. Musin, and R. Z. Musin, Synthesis, 2010, 3268; CrossRef d) X. K. Wee, W. K. Yeo, B. Zhang, V. B. C. Tan, K. M. Lim, T. E. Tay, and M.-L. Go, Bioorg. Med. Chem., 2009, 17, 7562. CrossRef
24. a) A. M. Taylor, R. A. Altman, and S. L. Buchwald, J. Am. Chem. Soc., 2009, 131, 9900; CrossRef b) L. Wang, Y. Su, X. Xu, and W. Zhang, Eur. J. Org. Chem., 2012, 6606.
25. a) Y.-M. Li, X.-H. Wei, and S.-D. Yang, Chem. Commun., 2013, 49, 11701; CrossRef b) L. Cheng, L. Liu, D. Wang, and Y.-J. Chen, Org. Lett., 2009, 11, 3874. CrossRef
26. a) T. Kato, A. Inada, Y. Morita, and H. Miyamae, Chem. Pharm. Bull., 1985, 33, 5270; CrossRef b) R. Underwood, K. Prasad, O. Repic, and G. E. Hardtmann, Synth. Commun., 1992, 22, 343. CrossRef
27. a) B. M. Trost and Y. Zhang, Chem. Eur. J., 2011, 17, 2919; b) Q.-S. Yu, W. Luo, H. W. Holloway, T. Utski, T. A. Perr, D. K. Lahiri, N. H. Greig, and A. Brossi, Heterocycles, 2003, 61, 529. CrossRef
28. E. Glamkowski and B. E. Kurys, Can. Pat. Appl., 1991, CA 2029265 A1 19910504.
29. a) M. Mori, S. Kudo, and Y. Ban, J. Chem. Soc., Perkin Trans. 1, 1979, 771; CrossRef b) T. Nishida, Y. Tokuda, and M. Tsuchiya, J. Chem. Soc., Perkin Trans. 2, 1995, 823; CrossRef c) M. Kihara, Y. Iwai, and Y. Nagao, Heterocycles, 1995, 41, 2279. CrossRef
30. a) L. M. Repka, J. Ni, and S. E. Reisman, J. Am. Chem. Soc., 2010, 132, 14418; CrossRef b) J. E. Spangler and H. M. L. Davies, J. Am. Chem. Soc., 2013, 135, 6802; CrossRef c) J. Ni, H. Wang, and S. E. Reisman, Tetrahedron, 2013, 69, 5622; CrossRef d) Y.-M. Su, Y. Hou, F. Yin, Y.-M. Xu, Y. Li, X. Zheng, and X.-S. Wang, Org. Lett., 2014, 16, 2958. CrossRef